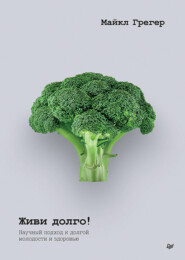
Полная версия:
Живи долго! Научный подход к долгой молодости и здоровью
Американские[3486] и европейские[3487] врачи рекомендуют снизить потребление кофеина. Это вполне логично. Кофеин является легким диуретиком, особенно в дозах, содержащихся в двух-трех чашках кофе, хотя ежедневное употребление кофе может вызвать привыкание, и эффект снизится[3488]. Удивительно, но метаанализ обсервационных исследований не выявил никакой связи между недержанием мочи и употреблением кофе или кофеина вообще[3489]. В двух из четырех интервенционных исследований, посвященных снижению потребления кофеина, было обнаружено уменьшение частоты мочеиспусканий (а в двух других – отсутствие заметных эффектов), но только в двух из семи подобных исследований, в которых рассматривались эпизоды недержания мочи, была выявлена значительная польза отказа от кофе. Однако, опять же, что мешает попробовать?
Ограничение жидкости в целом может быть контрпродуктивным, поскольку более концентрированная моча может раздражать слизистую оболочку мочевого пузыря и, как это ни парадоксально, делать позывы к мочеиспусканию более частыми и срочными[3490]. И еще: я бы посоветовал отказаться от диетических напитков. Сравнительный анализ показал, что диетическая кола увеличивает частоту мочеиспускания значительнее, чем обычная кола. Исследователи обвинили в этом искусственные подсластители, основываясь на результатах исследований in vitro на мочевом пузыре крыс: было выявлено усиление мышечных сокращений[3491].
Тренировка мышцДля лечения недержания мочи могут быть назначены препараты, подавляющие сокращение мышц мочевого пузыря[3492]. Средний процент излечения составляет около 50 %, однако лекарства обладают солидным перечнем побочных эффектов, которые я упоминал выше[3493]. Это объясняет, почему только 14–35 % людей, которым назначены эти препараты, продолжают принимать их через год[3494]. Лекарств для лечения стрессового недержания мочи, одобренных FDA, не существует[3495], но при хирургическом вмешательстве процент излечения превышает 80 %[3496].
Как это ни удивительно, существует множество доказательств того, что системная (пероральная) терапия эстрогенами может усилить недержание[3497]. Например, в исследовании «Инициатива по охране здоровья женщин» (Women's Health Initiative) было выявлено, что у женщин, получавших эстрогены, вероятность развития стрессового недержания мочи в течение первого года была примерно в 2 раза выше, чем при приеме плацебо[3498]. Местные (вагинальные) эстрогены, по-видимому, все же помогают, снижая частоту случаев неконтролируемого выделения мочи[3499]. Тем не менее самое эффективное средство для лечения недержания мочи – не фармакологическое и не хирургическое[3500]. В 5 раз лучше, чем местные эстрогены, работают упражнения Кегеля для тазового дна.
В 1948 году доктор Арнольд Кегель опубликовал работу, в которой описал успешный метод лечения недержания мочи, заключающийся в тренировке мышц, идущих от лобковой кости спереди, вниз и вокруг нее до копчика сзади[3501]. Чтобы найти нужные мышцы, остановите мочеиспускание в середине потока. Клиника Майо предлагает представить, что вы сидите на шарике и пытаетесь приподнять его с помощью мышц влагалища[3502]. Сокращения в течение десяти секунд с последующим расслаблением не менее десяти секунд рекомендуется выполнять от тридцати до ста раз в день в течение не менее месяца, чтобы увидеть результаты[3503]. Дополнительным стимулом к продолжению занятий является улучшение оргазма и сексуального удовлетворения – приятный побочный эффект тренировки мускулатуры таза[3504].
После того как мышцы таза придут в норму, можно использовать технику «замри и сожми» для подавления потребности помочиться при наступлении настоятельной необходимости или перед чиханием[3505]. При недержании мочи этот метод можно сочетать с тренировкой мочевого пузыря, которая заключается в постепенном увеличении интервала между мочеиспусканиями: начать с одного часа и увеличивать время на полчаса в неделю до тех пор, пока вы не сможете ждать два с половиной – три часа между каждым походом в туалет[3506]. Метаанализ 31 исследования с участием более 1800 женщин с недержанием мочи в 14 странах показал, что вероятность излечения у тех, кто был включен в программу тренировок мышц тазового дна по методу Кегеля, в среднем в 5 раз выше, чем у контрольной группы, не тренировавшей мышцы (а у женщин со стрессовым недержанием – в 8 раз выше)[3507].
Польза растяжки
Физическая активность снижает риск недержания мочи, и это не связано исключительно с упражнениями для тазового дна. Существуют результаты единственного исследования влияния йоги на недержание[3508]. Подробности см. в ролике see.nf/yogatrials. Они выявили, что по сравнению с группой, выполнявшей неспецифические упражнения на растяжение и укрепление мышц, те, кто занимался йогой, получили значительное преимущество при стрессовом недержании мочи, но не при ургентном недержании[3509].
Увеличение предстательной железы
Урологические нарушения у пожилых мужчин чаще всего вызваны увеличением предстательной железы – заболеванием, известным как доброкачественная гиперплазия предстательной железы (ДГПЖ). Ею страдают миллионы мужчин в США[3510] – до половины из них к 50 годам и 80 % мужчин к 80 годам[3511], что делает ДГПЖ одним из наиболее распространенных заболеваний, поражающих мужчин в западной популяции[3512]. Предстательная железа мужчины окружает выход из мочевого пузыря, поэтому при чрезмерном росте она будет препятствовать нормальному оттоку мочи. Это может вызвать слабую или медленную струю мочи и недостаточное опорожнение мочевого пузыря, что приводит к частым походам в туалет. Более того, застой мочи в мочевом пузыре может стать питательной средой для инфекции.
Фармацевтические и хирургические подходыК сожалению, проблема усугубляется по мере того, как железа продолжает увеличиваться в размерах. Миллионы американских мужчин перенесли операцию по поводу ДГПЖ, миллиарды были потрачены на лекарства и биологически активные добавки[3513]. Современные препараты действенны, но польза от них снижается из-за побочных эффектов и частых случаев отказа пациентов от их приема[3514]. Среди таких эффектов – сексуальная дисфункция, высокодифференцированный рак простаты и депрессия. Неудивительно, что мужчины не любят эти препараты принимать[3515]! По данным исследования, в котором приняли участие более миллиона американских мужчин, продолжили прием лекарства в течение года всего 29 % пациентов[3516].
Сексуальные расстройства, связанные с применением лекарств для лечения ДГПЖ, включают импотенцию, снижение либидо, нарушение эякуляции и гинекомастию (увеличение мужской груди)[3517]. В 2021 году благодаря судебному иску информационного агентства Reuters были обнародованы внутренние документы компании Merck, производящей препарат Proscar. Оказывается, еще в 2009 году компания Merck знала о том, что ее препарат вызывает стойкую эректильную дисфункцию (даже после прекращения приема препарата), но внутренний отдел управления рисками Merck решил не распространять эту информацию[3518].
Это подводит нас к «золотому стандарту» лечения ДГПЖ – хирургическому вмешательству[3519]. Процедуры включают в себя множество различных методик с невинно звучащими аббревиатурами, такими как TUMT, TUNA и TURP. Т означает «трансуретральный», то есть хирург вводит внутрь и вверх по половому члену инструмент, называемый резектоскопом. TUMT – это трансуретральная микроволновая термотерапия (transurethral microwave thermotherapy), при которой врачи с помощью инструмента, похожего на антенну, прокладывают тоннель в половом члене и микроволнами выжигают ствол[3520]. TUNA – трансуретральная игольчатая абляция (transurethral needle ablation), которая предполагает выжигание парой нагретых игл. И это еще так называемые малоинвазивные методики[3521]. При стандартной процедуре, называемой TURP – трансуретральной резекции простаты (transurethral resection of the prostate), хирурги используют проволочную петлю для резекции тканей. Побочные эффекты включают «послеоперационный дискомфорт»[3522].
Должен быть способ лучше.
Гиперплазия предстательной железы не является неизбежнойБольшинство врачей могут считать, что ДГПЖ – это просто неизбежное следствие старения, поскольку это очень распространенное заболевание, но так было не всегда. Например, в 1920–1930-х годах медицинский колледж в Пекине сообщал, что ДГПЖ страдают не 80 % пациентов мужского пола, а за 15 лет было выявлено лишь около 80 отдельных случаев. Столь низкая распространенность как ДГПЖ, так и рака простаты в Китае и Японии в ретроспективе объясняется традиционной растительной диетой этих стран[3523]. Недавние исследования мужчин народа цимане – боливийских фермеров, ведущих натуральное хозяйство и традиционно питающихся такими крахмалистыми продуктами, как бананы[3524], показали, что запущенные случаи ДГПЖ у них практически не встречаются – это подтверждает тот факт, что заболевание не является неизбежным[3525].
Популяционные исследования показывают, что защитным фактором можно признать низкое потребление животного белка и высокое потребление фруктов и овощей[3526]. По сравнению с мужчинами, употребляющими мясо реже одного раза в неделю, у тех, кто ест мясо ежедневно, вероятность развития симптомов ДГПЖ была более чем в 2 раза выше[3527]. В подробном исследовании ученые обнаружили, что хуже всего на здоровье простаты влияет употребление мяса птицы и яиц, а также рафинированных зерновых, но не было выявлено никакой связи с красным мясом или молочными продуктами[3528]. Лук и чеснок значительнее других растительных продуктов снижают риск развития ДГПЖ[3529]. Также для снижения риска лучше приготовленные овощи, чем сырые, хороши и бобовые – фасоль, горох, чечевица и нут[3530]. Мужчины, употребляющие изофлавоны, содержащиеся всего в одной чашке соевого молока[3531], также меньше рискуют[3532]. Текстурированный растительный белок, известный как TVP, – это соевый продукт, часто используемый в вегетарианском чили и соусах для макарон. Хотя я предпочитаю менее обработанные соевые продукты, я бы рекомендовал этот тип TVP вместо TVP, используемого в урологии, что означает трансуретральную вапоризацию простаты[3533].
Растения и простатаВ книге «Не сдохни!» я подробно описал серию экспериментов, проведенных Дином Орнишем и его коллегами. Он добавлял кровь людей до и после применения растительной диеты к раковым клеткам в чашке Петри. Кровь мужчин, придерживающихся стандартной американской диеты, замедляла рост раковых клеток простаты на 9 %. Когда же мужчины в течение года придерживались растительной диеты, кровь, циркулирующая в их организме, подавляла рост раковых клеток на 70 %. Почти в 8 раз больше возможностей для борьбы с раком дает растительное, а не мясное меню[3534]. (Аналогичные исследования показали, что женщины, придерживающиеся растительной диеты, всего за 2 недели значительно усиливают свою защиту от рака молочной железы[3535].) А что, если провести такой же эксперимент с нормальными клетками простаты, которые разрастаются и препятствуют оттоку мочи?
Уже через 2 недели кровь мужчин, питающихся растительной пищей, приобрела способность подавлять аномальный рост нераковых клеток предстательной железы. Более того, эффект не исчезал с течением времени. Кровь людей, длительно употребляющих растительную пищу, сохраняла противораковую силу в течение 28 лет подряд. Таким образом, похоже, что при условии здорового питания темпы роста клеток предстательной железы будут снижаться и оставаться на должном уровне[3536]. Однако некоторые растения могут быть особенно полезны для простаты.
Ягоды со пальметто и добавка, которая действительно работает
Ягоды со пальметто[3537] – самая распространенная растительная добавка, используемая для лечения ДГПЖ[3538], но она не работает[3539]. Одной из добавок, которая может помочь в профилактике[3540] и лечении[3541] ДГПЖ, является витамин D. Подробная информация о них приведена в видео see.nf/saw.
Перейти на семенаЛьняное семя можно использовать для лечения ДГПЖ. Мужчины, принимавшие ежедневно около трех столовых ложек семян льна, испытывали облегчение, сравнимое с тем, которое достигается при приеме широко назначаемых лекарственных препаратов[3542], но без их побочных эффектов. Тыквенные семечки также работают[3543], как подробно описано в ролике see.nf/seeds, что позволило европейскому аналогу FDA сделать вывод о возможности их использования для «облегчения симптомов заболеваний мочевых путей, связанных с увеличенной простатой, после исключения врачом более серьезных заболеваний»[3544].
Ночные походы в туалет
Одним из самых неприятных симптомов ДГПЖ является ноктурия – частые позывы встать посреди ночи, чтобы помочиться[3545]. Здравый смысл подсказывает, что нужно просто стараться меньше пить перед сном, но, что примечательно, четкой связи между потреблением жидкости и ноктурией не существует[3546]. Одно исследование, в котором приняли участие около 150 мужчин, обнаружило корреляцию между частотой ноктурии и потреблением воды в ночное время, а также количеством воды, выпитой за 4 часа до сна[3547]. Однако в другом исследовании, в котором приняли участие более тысячи пожилых людей, не было обнаружено никакой связи между количеством выпитой перед сном жидкости и необходимостью неоднократно вставать для мочеиспускания[3548]. Я был удивлен, узнав, что ограничение количества жидкости никогда не подвергалось должным испытаниям. Затем было проведено исследование, в котором группе пожилых мужчин, встававших в среднем четыре раза за ночь, было предложено сократить ежедневное потребление жидкости с семи до пяти стаканов, и им удалось уменьшить количество ночных походов в туалет до трех[3549]. Но в этом и других подобных исследованиях[3550], [3551] не было контрольной группы, чтобы установить реальную причинно-следственную связь.
Еще сложнее заставить людей ограничить потребление натрия. В обзорах по ноктурии с заголовками «Что важнее – вода или соль?»[3552] отмечается, что потребление соли связано с частотой ноктурии[3553], предположительно из-за увеличения потребления жидкости, вызванного жаждой. Это привело к появлению рекомендаций по снижению потребления соли для контроля ноктурии, однако ограничение потребления соли трудно поддается изучению, поскольку его мало кто соблюдает[3554]. Однако можно сравнить изменение количества эпизодов ноктурии у тех, кто успешно сократил потребление соли, и у тех, кто этого не сделал. Но основе этого сравнения был сделан вывод, что даже сокращение потребления соли всего лишь на половину чайной ложки в день может уменьшить количество ночных эпизодов на 40–60 %[3555], [3556].
Вечернее потребление белка также способствует развитию ноктурии. Основным фактором, определяющим концентрацию мочи, является не натрий, а мочевина – продукт распада белков. Было обнаружено, что богатые белком ужины коррелируют с избыточным ночным выделением мочи. Это позволило сделать предположение, что «снижение вечернего потребления белка может быть эффективно при лечении ноктурии…»[3557].
Некоторые любят покислееКакие еще продукты питания могут помочь? Клюква когда-то использовалась коренными американцами для лечения болезней мочевыводящих путей[3558]. Клюква может успешно уменьшать простату грызунов на 33 %[3559], но итоги первого испытания на человеке, в котором проверялась эффективность сушеной клюквы (Vaccinium macrocarpon) у мужчин с симптомами заболеваний мочевых путей, были опубликованы только в 2010 году. Использовался обычный цельный клюквенный порошок. Значительное улучшение симптомов ДГПЖ, качества жизни и всех параметров мочеиспускания было отмечено при приеме примерно трех четвертей чайной ложки в день порошка клюквы[3560].
А если ограничиться четвертью или даже одной восьмой чайной ложки порошка? Обе эти дозы превзошли плацебо по снижению симптомов ДГПЖ[3561]. Исследователи использовали фирменную добавку, но поскольку это просто порошок из плодов клюквы, вы вполне можете купить его оптом, что гораздо дешевле, и добавлять в смузи или посыпать им овсяную кашу.
Пилотное исследование также показало, что клюква может предотвращать рецидивирующие инфекции мочевыводящих путей у пожилых мужчин с ДГПЖ, однако в контролируемом исследовании не было ни плацебо, ни даже рандомизированного распределения, что делает полученные результаты в лучшем случае гипотезами[3562].
Чеснок и помидорыА как насчет ягоды, которая немного вкуснее? Исследователи пробовали применять сок фиолетового винограда сорта Конкорд для лечения ДГПЖ, но он не показал никакой пользы[3563]. Если клюква – самая эффективная ягода, то что может быть самым эффективным овощем? В видео see.nf/garlictomatoes я рассматриваю испытания томатной пасты[3564] и экстракта чеснока[3565] для лечения ДГПЖ. К сожалению, оба исследования проводились по принципу «до и после» без контрольных групп, поэтому преимущества являются лишь предположением.
Сохранение кровообращения
Известный английский врач XVII века Томас Сиденхам сказал: «Мужчина стар настолько, насколько стары его артерии»[3566]. Женщины тоже, хотя, похоже, мало кто это признает. По данным общенационального опроса женщин США, большинство из них считают, что наибольший риск для их здоровья представляет рак. Только 13 % правильно определили в качестве главной угрозы сердечно-сосудистые заболевания, которые на самом деле являются первой по распространенности причиной смерти женщин (и мужчин[3567], и долгожителей любого пола[3568]). В период с 2009 по 2019 год опросы Американской кардиологической ассоциации отметили «тревожное снижение» доли женщин, считающих сердечно-сосудистые заболевания основной причиной своей смерти[3569].
В недавней редакционной статье журнала Aging Medicine авторы поэтически утверждали, что «кровеносный сосуд – это свеча жизни», и заявили, что «все болезни исходят из сосудов»[3570]. Существует даже микроциркуляторная теория старения, согласно которой потеря плотности кровеносных сосудов с возрастом – до 50 % в некоторых тканях, например в области мозга, – может способствовать ухудшению состояния органов, поскольку нарушается удаление отходов и доставка кислорода и питательных веществ[3571]. Можно сказать, что кровь приносит нам жизнь.
Как сохранить молодость сердца?
Способность наших кровеносных сосудов к самовосстановлению зависит от эндотелиальных прогениторных клеток, которые образуются из стволовых клеток в костном мозге и заделывают все дыры в эндотелии – внутренней оболочке сосудов, обеспечивающей беспрепятственное движение крови[3572]. Смотрите в видео see.nf/epc демонстрацию силы эндотелиальных прогениторных клеток[3573] и того, что мы можем сделать для увеличения их количества и функциональности. Стоит начать с того, чтобы избегать даже пассивного курения сигарет[3574] и регулярно заниматься аэробными физическими упражнениями, что[3575] считается лучшим средством для профилактики и лечения старения артерий[3576]. А что насчет диеты?
Рандомизированное контролируемое исследование показало, что снижение потребления насыщенных жиров (в основном сливочного масла) значительно увеличивает количество эндотелиальных клеток-предшественников[3577]. Это согласуется с результатами исследования на бабуинах, показавшего, что даже несколько недель диеты с высоким содержанием холестерина и жиров могут вызвать резкое преждевременное старение эндотелиальных клеток[3578]. А вот в список продуктов, которые увеличивают количество циркулирующих эндотелиальных прогениторных клеток, вошли ягоды[3579], лук[3580] и зеленый чай[3581]. Диета, полностью состоящая из цельной растительной пищи, не только привела к увеличению количества эндотелиальных прогениторных клеток, но и улучшила функцию эндотелия, а также снизила уровень холестерина ЛПНП[3582].
Нормальный уровень холестерина – это смертельно опасный уровень холестерина
Научные консенсусные группы, созданные на протяжении десятилетий, установили, «вне всяких сомнений», что снижение уровня холестерина ЛПНП уменьшает риск сердечных приступов[3583]. Они безоговорочно подтверждают, что ЛПНП вызывают причину смерти номер один – сердечно-сосудистые заболевания. Доказательная база включает сотни исследований с участием буквально миллионов людей[3584]. Другими словами, «[это] холестерин, дурачок», – сказал главный редактор American Journal of Cardiology[3585] Уильям Клиффорд Робертс. Его CV насчитывает более ста страниц, а в рецензируемой медицинской литературе он опубликовал около 1700 статей[3586]. Да, существует не менее десяти традиционных факторов риска развития атеросклероза, но, как отмечает доктор Робертс, для прогрессирования заболевания необходим всего один – повышенный уровень холестерина[3587]. Все остальные факторы, такие как курение, повышенное артериальное давление, диабет, гиподинамия и ожирение, лишь усугубляют ущерб, наносимый высоким уровнем холестерина[3588].
Фух! – с облегчением выдохнете вы, когда получите результат анализа крови и врач скажет, что уровень холестерина у вас «в норме». Но подождите. Нормальный уровень холестерина в обществе, где считается нормальным умереть от сердечного приступа, – это не повод для радости. Учитывая, что сердечно-сосудистые заболевания являются главной причиной смерти мужчин и женщин, мы определенно не хотим иметь нормальный уровень холестерина. Мы хотим иметь оптимальный уровень – не по произвольным лабораторным стандартам, а оптимальный для здоровья человека.
Уровень холестерина ЛПНП, считающийся нормальным, вызывает образование атеросклеротических бляшек в наших артериях[3589] даже у тех, кто, по современным стандартам, не находится в зоне риска: у кого артериальное давление менее 120 на 80, нормальный уровень сахара в крови и общий холестерин менее 200[3590]. Если бы вы пришли к врачу с такими показателями, то получили бы золотую звезду. Но когда с помощью ультразвука и компьютерной томографии удалось заглянуть внутрь тела пациентов с такими показателями, у 38 % из них были обнаружены атеросклеротические бляшки. Возможно, те цифры не так уж оптимальны.
Может быть, нам следует определять уровень холестерина ЛПНП как оптимальный только тогда, когда он больше не вызывает заболеваний[3591]? (Вот это концепция!) Как бы нам это выяснить?
Когда более тысячи мужчин и женщин в возрасте 40 лет прошли сканирование, у большинства из них с «нормальным» уровнем ЛПНП менее 130 был выявлен атеросклероз. Атеросклеротические бляшки не обнаруживались только при снижении уровня ЛПНП примерно до 50 или 60[3592], что, как оказалось, соответствует уровню, который был у большинства людей до нынешнего драматического изменения рациона питания[3593]. У основной массы взрослого населения планеты уровень ЛПНП составлял около 50 мг/дл. Таким образом, средние значения, признаваемые нормальными, рассчитываются на основе данных больного общества[3594]. Но нам нужен нормальный для здорового человека уровень холестерина, который считается равным 30–70 мг/дл (или 0,8–1,8 ммоль/л)[3595].
Хотя уровень ЛПНП в этом диапазоне может показаться чрезмерно низким по современным американским меркам, он как раз и является нормальным для людей, ведущих тот образ жизни и питающихся по той диете[3596], к которой наши предки генетически приспособились за миллионы лет: диета, в основе которой лежит цельная растительная пища[3597]. Уровень ЛПНП, на который был рассчитан наш организм, составляет менее половины от того, что сегодня считается «нормой»[3598], так что неудивительно, что мы переживаем пандемию атеросклеротических заболеваний сердца.
Почему-то в медицине существует тенденция ориентироваться на небольшие изменения факторов риска[3599], в то время как ее целью должно быть не просто снижение риска, а предотвращение образования бляшек[3600]. В таком случае, насколько низко мы должны опускаться[3601]?
Один из известных профессоров сосудистой биохимии отмечает: «В свете последних данных, полученных в ходе исследований преимуществ и рисков глубокого снижения уровня холестерина ЛПНП, ответом на вопрос “Насколько низко мы должны опуститься?”, вероятно, должен быть прямолинейный ответ: “Настолько низко, насколько это возможно!”»[3602] Однако важно, как мы этого добьемся. Низкий уровень действительно может быть лучше, но если мы снижаем уровень ЛПНП с помощью лекарств, то необходимо соизмерять пользу и риск побочных эффектов лекарств[3603].
Да, было бы здорово, если бы холестерин у всех был ниже, но сами препараты имеют контрриски[3604]. Врачи стремятся использовать статины в максимально возможной дозе, чтобы добиться значительного снижения уровня холестерина ЛПНП без увеличения риска повреждения мышц, которое могут вызвать эти препараты[3605]. Статины также повышают риск развития диабета 2-го типа[3606]. Однако если для снижения уровня холестерина сделать образ жизни более здоровым, то можно получить только преимущества[3607], включая значительное снижение риска развития диабета[3608]. Но удастся ли добиться достаточно низкого уровня ЛПНП только с помощью диеты?