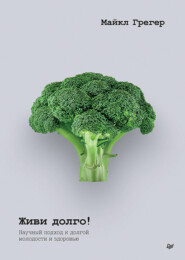
Полная версия:
Живи долго! Научный подход к долгой молодости и здоровью
Несмотря на более чем сотню рандомизированных клинических исследований различных средств для лечения запоров[3372], до сих пор отсутствуют полноценные данные о безопасности и эффективности слабительных средств для пожилых людей[3373]. Например, препарат для размягчения стула, по-видимому, неэффективно устраняет запоры, несмотря на то что он часто используется в качестве одного из наиболее распространенных безрецептурных средств[3374]. Стимулирующие слабительные средства разрешены только для краткосрочного применения (менее четырех недель), но, к сожалению, широко распространено их длительное применение в течение месяцев и даже лет[3375]. Биопсия, взятая у лиц, длительно принимающих стимулирующие слабительные, показывает, что нервы, иннервирующие толстую кишку, могут быть «серьезно повреждены»[3376].
Безрецептурным слабительным средством с наилучшими показателями безопасности[3377] и эффективности[3378], вероятно, является полиэтиленгликоль – не путать с этиленгликолем, или антифризом, который может привести к летальному исходу при попадании в организм[3379].
Большинство лекарственных средств, применяемых в настоящее время для лечения запоров, в целом безопасны при соблюдении рекомендаций, однако их эффективность оставляет желать лучшего[3380]. По результатам опроса более тысячи мужчин и женщин, страдающих хроническими запорами (а большинство из них принимает безрецептурные препараты), отметили, что лекарства практически не оказывают никакого воздействия на запоры (62 %) или на симптомы в кишечнике, связанные с запорами (78 %)[3381]. Должен быть лучший способ.
Плавные движенияСуществует множество подходов к лечению запоров, например завтракать горячим напитком, чтобы запустить гастроколический рефлекс[3382]. Но святая троица, о которой чаще всего говорят врачи, – это пищевые волокна, жидкость и физические упражнения[3383]. Что касается физических упражнений, то популяционные исследования не показывают четкой связи между запорами и физической активностью после учета других факторов, например потребления клетчатки, но нельзя знать наверняка, пока не проведешь тест[3384].
Малоподвижность, по-видимому, действительно замедляет работу кишечника. Когда активные пожилые люди стали вести сидячий образ жизни, снизив количество шагов в день с 13 000 до 4000, время транзита по толстой кишке у них в течение 2 недель увеличилось почти вдвое [3385]. (Время прохождения пищи от рта до ануса можно измерить, просто съев немного свеклы[3386].) И наоборот, даже умеренная физическая активность уменьшает симптомы вздутия живота. Но что насчет запоров?
На сегодняшний день проведено 9 рандомизированных контролируемых исследований физических упражнений при запорах у взрослых. Было показано, что даже умеренные аэробные упражнения, такие как ходьба в течение двадцати минут в день, способны ослабить симптомы легкого запора[3387], хотя это не было проверено в отношении тяжелых запоров[3388]. А помогает ли жидкость?
В ролике see.nf/mineralwater я рассматриваю все интервенционные исследования по увеличению потребления жидкости в борьбе с запорами, включая риски и преимущества использования соли Эпсома (сульфат магния) и клизм с фосфатом натрия. Я пришел к выводу, что лучшим средством от запора может быть устранение его причины путем употребления клетчатки, которая считается первейшим средством в лечении запоров[3389].
Заболевание, связанное с дефицитом клетчаткиЗапор можно считать болезнью, связанной с дефицитом питательных веществ, и этим питательным веществом является клетчатка[3390]. Менее 3 % американцев употребляют ее в том объеме, который соответствует рекомендованной минимальной суточной норме потребления клетчатки, что означает, что американцы не едят достаточно цельной растительной пищи – единственной, где клетчатка содержится в изобилии[3391]. Неудивительно, что у тех, кто придерживается строго растительной диеты, вероятность ежедневного опорожнения кишечника в 3 раза выше[3392]. Если бы половина взрослого населения США потребляла дополнительно 3 г клетчатки в день – четверть чашки бобов или чашку овсянки, – мы могли бы потенциально сэкономить миллиарды на медицинских расходах, необходимых для лечения запоров, поскольку известно, что в масштабах населения ежедневное увеличение количества пищевых волокон всего на 1 г приведет к снижению распространенности запоров примерно на 2 %[3393]. Трудно создать плацебо из толченой пшеницы, но можно доказать причинно-следственные связи с помощью рандомизированных двойных слепых плацебо-контролируемых исследований с использованием пищевых добавок с клетчаткой.
Добавки с клетчаткойДобавки с клетчаткой[3394], являющиеся наиболее распространенным средством лечения запоров, рекомендуются американскими, европейскими и мировыми руководствами в качестве самых эффективных[3395]. Растворимые неферментируемые волокна, такие как псиллиум (также известный как исфагула), считаются наиболее подходящим выбором[3396]. Псиллиум задерживает воду в кишечнике, увеличивая содержание воды и объем стула для облегчения дефекации, но именно по этой причине важно принимать его согласно инструкции и с достаточным количеством жидкости[3397]. В противном случае псиллиум может вызвать кишечную непроходимость[3398]. Более подробную информацию об эффективности и потенциальных дополнительных преимуществах этих средств можно найти в ролике see.nf/fibersupplements.
Клетчатка из продуктов питания, а не из добавокЛучше всего получать клетчатку не из аптеки, а из продуктового магазина, в первую очередь из отдела бакалеи. Помимо регулярной работы кишечника высокое потребление пищевых волокон связано со снижением риска сердечно-сосудистых заболеваний[3399], [3400], рака[3401], ожирения[3402], диабета[3403], депрессии[3404] и преждевременной смерти в целом[3405]. Каждые 7 г клетчатки, получаемой ежедневно, коррелируют с 9 %-ным снижением риска сердечно-сосудистых заболеваний – убийцы номер один[3406]. Так неужели 77 г в день снизят риск на 99 %? Примерно столько клетчатки потребляли в Уганде[3407] – стране, где ишемическая болезнь сердца практически не встречалась[3408].
Болезни сердца были настолько редки среди тех, кто питался традиционной растительной пищей в Уганде, что в качестве сенсации однажды была опубликована статья с названием «Случай ишемической болезни сердца у африканца»[3409]. После 26 лет медицинской практики в Восточной Африке врачи наконец-то зарегистрировали первый такой случай. (Пациентом был судья, который придерживался «частично вестернизированной диеты», в которой продукты, не содержащие клетчатки, такие как мясо, молоко и яйца, вытеснили часть растительной пищи из его традиционного рациона). Разумеется, после вестернизации пищевых привычек на всем континенте сердечно-сосудистые заболевания стали основной причиной смерти, превратившись из практически несуществующей чуть ли не в эпидемию[3410].
Редкость типичных для Запада заболеваний в сельских районах Африки к югу от Сахары привела к появлению гипотезы о пищевых волокнах, согласно которой диеты, основанные на употреблении цельной растительной пищи, защищают от хронических заболеваний благодаря содержанию в них клетчатки[3411]. Предсказуемо возник многомиллиардный рынок пищевых добавок с клетчаткой[3412]. Однако есть одна проблема. Они не работают[3413].
Добавки с клетчаткой могут быть полезны при запорах, но, по-видимому, они не обеспечивают никаких других преимуществ, связанных с хроническими заболеваниями. Действительно, исследования, связывающие снижение риска заболеваний и смерти с высоким потреблением клетчатки, относятся исключительно к клетчатке из пищи, а не к ее изолятам или добавкам[3414]. Возможно, это связано с тем, что клетчатка является маркером потребления здоровой цельной растительной пищи, или с ее ролью «курьера»[3415].
Основная роль пищевых волокон, вероятно, заключается в инкапсуляции питательных веществ для доставки их в микробиом нашего кишечника. Волокно – это кирпичик, из которого построены клеточные стенки растений, и эти клеточные стенки выступают в качестве неперевариваемых физических барьеров. Поэтому когда вы едите структурно неповрежденные растительные продукты, часть питательных веществ остается в них. Вы можете жевать сколько угодно, но в итоге такие питательные вещества, как крахмал, все равно будут полностью окружены клетчаткой, доставляя питание вашей полезной флоре. Затем она получает возможность питаться не только клетчаткой, но и всеми продуктами, которые та обволакивает. А вот добавки с клетчаткой, такие как псиллиум, не доставляют бактериям никакой пользы и даже сами не ферментируются, поэтому мы можем лишиться всех тех вспомогательных преимуществ, которые дает микробиому диета с высоким содержанием клетчатки[3416].
Лен и рожь
Молотые льняные семена – прекрасный цельный источник клетчатки[3417]. В течение 12 недель больные сахарным диабетом, страдающие запорами, получали печенье, содержащее около столовой ложки измельченных семян льна в день, или плацебо без льна. Лен не только ослабил симптомы запора, такие как боль при дефекации, напряжение и твердый стул, но и, по сравнению с плацебо, привел к снижению веса на 8 килограммов, уровня сахара в крови на 25 пунктов, уровня HbA1c на 1,8 % и уровня холестерина ЛПНП на 17 пунктов[3418]. Для сравнения льняного семени и псиллиума была добавлена вторая группа, которая употребляла печенье, содержащее 10 г псиллиума. Льняное семя все равно победило, обойдя псиллиум по показателям избавления от запоров, снижения веса, уровня сахара в крови и холестерина[3419]. (Кроме того, лен в 4 раза дешевле, чем даже дженерик псиллиума). Льняное семя также сравнивали непосредственно со слабительным лактулозой – и лен вновь победил, увеличив частоту опорожнения кишечника с двух до семи раз в неделю (при использовании лактулозы – шесть раз в неделю)[3420].
Также были проведены испытания ржаного хлеба с высоким содержанием клетчатки: 5 г клетчатки на ломтик; участникам исследования было предложено съедать по восемь ломтиков в день. По сравнению с белым хлебом, содержащим всего 1 г клетчатки на ломтик, ржаной хлеб с высоким содержанием клетчатки «явно уменьшил запор», увеличив частоту опорожнения кишечника, комфортность, мягкость стула и время прохождения кала по кишечнику. Однако в группе, употреблявшей ржаной хлеб, наблюдались повышенный метеоризм и вздутие живота, особенно в первую неделю, но по мере адаптации микрофлоры кишечника и установления баланса газообразующих и газоутилизирующих бактерий эти симптомы уменьшались[3421]. (В качестве примечания следует отметить, что термин «старые пердуны» может быть не только уничижительным, но и ошибочным. По результатам опроса 16 тысяч американцев, пожилые люди, как правило, страдают этим реже, чем представители более молодых возрастных групп[3422].)
Чернослив и манго
Несколько десятилетий назад в журнале Geriatric Nursing была опубликована статья «Специальный рецепт для устранения запоров», в которой подтверждалась эффективность ежедневного приема специального состава. Базовый рецепт состоял из двух чашек яблочного пюре, двух чашек необработанных пшеничных отрубей и одной чашки стопроцентного сливового сока. Такое блюдо выдавалось постояльцам домов престарелых в маленьких медицинских стаканчиках[3423]. Оно стоит в 2 раза дешевле псиллиума[3424]. Но что говорят научные исследования о черносливе?
В ролике see.nf/prune приводится их обзор. По сути, десять штук чернослива в день превзошли псиллиум в сравнительном тесте, где оценивались частота и консистенция стула, – чернослив увеличил регулярность с двух опорожнений кишечника в неделю до четырех; в группе, получавшей псиллиум, дефекация происходила три раза в неделю[3425]. (Для сравнения: у тех, кто придерживается растительной диеты, в среднем бывает около 11 опорожнений кишечника в неделю[3426].) С известной оговоркой: исследование финансировалось Калифорнийским советом по сухофруктам – исследователи предложили «рассматривать чернослив в качестве эффективной терапии при хронических запорах»[3427].
Инжир не показал никаких значимых результатов[3428], но исследование, проведенное при финансовой поддержке Mango Board (Национальным советом манго), показало, что свежие манго также смогли превзойти псиллиум. Мужчины и женщины, страдающие хроническими запорами, были рандомизированы для употребления либо одного манго в день, либо эквивалентного количества клетчатки в виде псиллиума (одна чайная ложка в день). По истечении одного месяца оказалось, что манго не только лучше помогало избавиться от запоров, но и оказывало значительное противовоспалительное действие, снижая уровень IL-6 в крови более чем на 20 %[3429].
На основе исследований микробиома мышей было высказано предположение, что это связано с пребиотическим эффектом мякоти манго [3430], что было подтверждено на людях в 2020 году, когда ученые обнаружили, что одно манго в день в течение 8 недель значительно увеличивает численность Lactobacillus – полезных бактерий в нашем кишечнике[3431].
Колоректальный рак
Колоректальный рак (рак толстой и прямой кишки) ежегодно уносит 50 тысяч жизней в США и является одним из наиболее часто диагностируемых видов рака. В течение жизни вероятность развития этого заболевания у среднестатистического человека составляет 1: 20[3432]. К счастью, это один из наиболее излечимых видов рака, если его обнаружить на ранней стадии, а плановое обследование позволяет врачам выявлять и удалять рак до того, как он начнет давать метастазы. Только в США насчитывается более миллиона человек, переживших колоректальный рак, а для тех, кому диагноз был поставлен до того, как рак распространился за пределы толстой кишки, пятилетняя выживаемость составляет около 90 %[3433]. Однако на ранних стадиях колоректальный рак редко вызывает симптомы. Если его не обнаружить до более поздней стадии, лечение будет менее эффективным и более сложным. В книге «Не сдохни!» я рекомендую проходить скрининг на колоректальный рак, начиная с 50 лет[3434], но сорок пять – это, возможно, новые пятьдесят в данном контексте.
В 2018 году Американское онкологическое общество стало первой крупной организацией, предложившей лицам со средним риском развития колоректального рака начинать скрининг не с пятидесяти, а с 45 лет[3435]. Однако Американский колледж врачей продолжал настаивать, что начинать скрининг следует с 50 лет, а Рабочая группа по профилактике заболеваний США, наиболее авторитетная американская организация, занимающаяся разработкой рекомендаций, о которой я упоминал ранее, обсудив все «за» и «против» и приняв во внимание участившиеся в последнее время случаи выявления опухолей на поздних стадиях у людей в возрасте до 40 лет[3436], пришла к выводу, что оптимальный возраст начала скрининга колоректального рака, вероятно, – 45 лет[3437].
На долю «раннего» колоректального рака, диагностируемого в возрасте до 50 лет, пока приходится лишь около 10 % случаев, однако с середины 1990-х годов этот показатель увеличился на 50 %[3438]. Нынешний уровень заболеваемости среди 45-летних сопоставим с уровнем заболеваемости среди 50-летних в 1990-е годы, когда были даны первоначальные рекомендации начинать скрининг с 50 лет[3439]. Отчасти этот рост связывают с растущей распространенностью ожирения[3440], хотя, возможно, свою роль сыграло и чрезмерное использование антибиотиков у детей[3441]. Особому риску подвергаются афроамериканские мужчины[3442], о чем свидетельствует трагическая смерть актера Чедвика Боузмана от колоректального рака в возрасте 43 лет. По сравнению с белыми американцами риск смерти от колоректального рака у чернокожих американцев на 40 % выше[3443], однако при опросе большинство из них ошибочно считают, что риск заболевания у них ниже[3444]. Американская коллегия врачей рекомендовала афроамериканцам начинать скрининг с 40 лет[3445].
Проведение колоноскопииПо данным USPSTF, существует шесть приемлемых стратегий скрининга рака толстой кишки. Начиная с 45-летнего возраста, каждый человек должен проходить одну из следующих скрининговых процедур: колоноскопию раз в 10 лет; ежегодное исследование кала на скрытую кровь; исследование кала на ДНК-маркеры раз в 1–3 года (например, с помощью Cologuard); «виртуальную» колоноскопию с использованием рентгеновской компьютерной томографии; гибкую сигмоидоскопию либо раз в 5 лет либо раз в 10 лет с ежегодным тестированием на ДНК-маркеры[3446].
Почему почти все американские врачи рекомендуют колоноскопию[3447], в то время как в большинстве других стран мира предпочтительным методом скрининга является неинвазивное исследование кала[3448]? Возможно, это связано с тем, что большинство врачей, практикующих в других странах мира, не получают зарплату исходя из количества процедур[3449]. Как сказал один американский гастроэнтеролог, «колоноскопия… это курица, несущая золотые яйца»[3450]. Для принятия решения обратитесь к моему подробному описанию рисков и преимуществ колоноскопии в книге «Не сдохни!». В конце концов, лучший метод скрининга – это тот, который вы действительно будете делать[3451].
Профилактика колоректального ракаКак ни странно, одной из отрицательных сторон скрининга является так называемый эффект сертификата здоровья, когда люди, прошедшие скрининг, считают себя здоровыми и у них снижается стимул к ведению здорового образа жизни[3452]. Действительно, исследование показало, что те, кому был проведен скрининг колоректального рака, в итоге снизили потребление фруктов и овощей[3453], что в конечном счете может перевесить положительный эффект от скрининга[3454]. Решением проблемы может стать консультирование по вопросам образа жизни в рамках скринингового визита[3455].
Регулярные скрининги для выявления колоректального рака, безусловно, имеют смысл, но еще лучше предотвратить болезнь. Риск рака толстой кишки можно снизить примерно на 30 %, регулярно проходя процедуры колоноскопии и сигмоидоскопии[3456], но снизить риск на 71 % можно с помощью простых изменений в питании и образе жизни: например, уменьшив потребление мяса[3457]. Чтобы выявить наиболее значимые факторы образа жизни, ученые исследовали, где уровень заболеваемости раком толстой кишки самый низкий.
В США рак толстой кишки остается вторым по распространенности онкологическим заболеванием, а в сельских районах Африки он встречается в 10 раз реже. Исследования мигрантов показывают, что различия в глобальных показателях не являются генетическими, поскольку иммигрантам может потребоваться всего одно поколение, чтобы войти в статистику заболеваемости раком толстой кишки, характерную для их новой страны. Наиболее вероятной причиной считаются изменения в рационе питания, однако при переходе из одной культуры в другую происходят самые разные изменения: от распространенности курения до воздействия химических веществ, инфекций и антибиотиков[3458]. Невозможно понять, виновата ли диета, пока не проведешь тест.
Посмотрите мой видеоролик see.nf/switchdiets, чтобы узнать, как работает толстый кишечник афроамериканцев, следующих традиционной африканской диете с высоким содержанием клетчатки, и толстый кишечник коренных африканцев, перешедших на стандартную американскую диету[3459]. Короче говоря, как выразился ведущий исследователь, «измените свой рацион – измените риск развития рака!»[3460].
По результатам исследований, в которых приняли участие более 3 миллионов человек, можно сделать вывод, что растительная диета ассоциируется со значительно более низким числом опухолей пищеварительного тракта, включая рак толстой и прямой кишки[3461]. Учитывая «потрясающе положительный эффект» употребления цельной растительной пищи, снижающего риск развития рака, один из ученых заключил: «Хотя было бы нереалистично ожидать быстрых и глубоких изменений в образе жизни широких слоев населения, отрадно, что у нас есть разумные и эффективные рекомендации для тех, кто готов предпринять шаги, необходимые для здорового долголетия»[3462].
Недержание мочи
Мы рассмотрели все тонкости сохранения функции кишечника. А что же с мочевым пузырем? Недержание мочи определяется как любое непроизвольное подтекание мочи[3463]. Существует два типа недержания: ургентное недержание, определяемое как непроизвольное мочеиспускание, связанное с внезапным сильным желанием помочиться, и стрессовое недержание, когда, например, чихание провоцирует вытекание урины[3464]. Женщины страдают от этой проблемы в 2–3 раза чаще, чем мужчины, особенно с возрастом[3465]. Связано это с тем, что с годами количество мышечных волокон в сфинктере уретры у женщин уменьшается[3466]. Снижается способность стареющих почек концентрировать мочу, развивается синдром раздраженного мочевого пузыря, уменьшается его емкость, и он реже опорожняться полностью. Все это может осложняться нарушением ощущения наполненности мочевого пузыря[3467].
Около трети опрошенных в США людей верят в миф о том, что недержание – это неизбежное последствие старения, и с возрастом оно действительно становится все более распространенным[3468]. В возрасте старше 70 лет им могут страдать 40 % женщин[3469], а в возрасте старше 80 лет эта цифра может возрасти до 55 %[3470]. В любом возрасте недержание мочи ассоциируется с ухудшением качества жизни[3471]. Что мы можем сделать для его профилактики и лечения?
Диета при недержанииРаспространенность среди женщин недержания мочи связана с родами. По сравнению с кесаревым сечением, вагинальные роды могут в 3 раза увеличить риск развития недержания мочи в будущем[3472]; полагают, что это вызвано растяжением мышц и нервов в процессе родов[3473]. Особенно это проявляется при поздних родах[3474].
У женщин с ожирением вероятность развития тяжелой формы недержания мочи в 3 раза выше, чем у женщин со здоровым весом[3475]. Это может быть связано с повышением внутрибрюшного давления, передающегося мочевому пузырю[3476]. Помимо наблюдений, интервенционные исследования показывают, что помочь может даже умеренное снижение веса[3477]. Например, в исследовании, проведенном в рамках Программы по уменьшению недержания мочи с помощью диеты и физических упражнений (Program to Reduce Incontinence by Diet and Exercise, PRIDE), сотни женщин с избыточным весом и ожирением были разделены на две группы: одна следовала программе снижения веса, другая была контрольной: получала только общие образовательные курсы по вопросам здоровья. Участницы программы снижения веса потеряли в среднем на 14 килограммов больше, чем контрольная группа, и испытывали значительно меньше эпизодов недержания. По истечении 6 месяцев частота случаев недержания у женщин из группы снижения веса сократилась более чем наполовину – на 61 %; у контрольной группы результат был 34 %[3478].
Другая проблема, вне зависимости от наличия или отсутствия недержания мочи, – это гиперактивный мочевой пузырь; определяется как срочность мочеиспускания, часто сопровождающаяся его учащением. Каждая третья женщина сталкивается с гиперактивным мочевым пузырем в течение жизни, причем с возрастом распространенность этого увеличивается[3479]. Однако рандомизированное двойное слепое плацебо-контролируемое исследование показало, что для облегчения состояния может потребоваться всего 1/2 г сушеного клюквенного порошка. Лекарства, расслабляющие мочевой пузырь, представляют собой многомиллиардную индустрию[3480], но при этом могут сократить количество мочеиспусканий в среднем на 16 раз в месяц, то есть меньше примерно на одно мочеиспускание в два дня[3481]. Однако менее четверти чайной ложки клюквенного порошка действуют почти в 4 раза лучше, в результате чего количество походов в туалет в день сокращается почти на два. И не забудьте, что у таблеток есть побочные эффекты, среди которых сухость во рту, запоры, седативный эффект, нарушение когнитивных функций, учащенное сердцебиение, задержка мочи и нарушения зрения, из-за чего почти две трети пациентов прекращают прием препарата[3482].
Популярная пресса советует сократить потребление «раздражителей мочевого пузыря», таких как острая, соленая и кислая пища. Опубликованных данных, подтверждающих эту рекомендацию, нет, но прелесть безопасных и простых диетических изменений заключается в том, что вы можете попробовать и посмотреть, почувствуете ли себя лучше[3483]. В лонгитюдном исследовании, в котором приняли участие более чем 5000 женщин, было обнаружено, что только два компонента рациона питания значимо связаны со стрессовым недержанием: это насыщенные жиры и холестерин[3484], хотя они могут быть просто косвенными показателями более нездорового питания и/или образа жизни. Связь между потреблением фитоэстрогенов (например, сои или льняного семени) и симптомами недержания мочи, по-видимому, отсутствует[3485]. А как насчет сокращения потребления кофе?