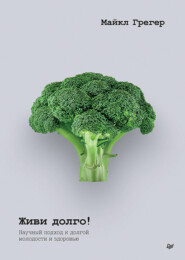
Полная версия:
Живи долго! Научный подход к долгой молодости и здоровью
Спросите у ведущих специалистов страны, к какому уровню холестерина они стремятся, и, скорее всего, вы услышите что-то вроде: ЛПНП ниже 70 или около того[3609]. Простое сокращение потребления насыщенных и трансжиров, содержащихся в мясе, молочных продуктах и вредной пище, а также снижение потребления пищевого холестерина, главный источник которого – яйца, вряд ли поможет большинству людей достичь этой цели[3610]. Однако у тех, кто питается полностью растительной пищей, уровень ЛПНП может быть в среднем именно таким низким[3611]. Неудивительно, что растительная диета – единственная модель питания, которая, как было доказано, способна обратить вспять прогрессирование ишемической болезни сердца[3612].
Давление в норме
Аналогичная парадигма «смертельной нормы» существует и в отношении артериального давления. Известно, что основным фактором риска смерти в США является американская диета, на втором месте – табак, но убийцей номер три является высокое артериальное давление, также известное как гипертония[3613]. Оно настолько смертельно опасно, что повышает риск смерти от множества различных заболеваний – от болезней сердца и инсульта до сердечной и почечной недостаточности[3614].
Посмотрите мой видеоролик see.nf/bloodpressure, чтобы ознакомиться с изменением рекомендаций. Риск умереть от инсульта или сердечных заболеваний экспоненциально возрастает по мере повышения артериального давления сверх 110/70[3615]. Однако принудительное снижение давления с помощью лекарств приведет к печальным последствиям. Например, если людям, относящимся к группе повышенного риска, назначить достаточно высокие дозы лекарств, чтобы снизить давление даже до верхней цифры 120, можно будет ежегодно предотвращать более 100 000 смертей и 46 000 случаев сердечной недостаточности. В то же время это может привести к 43 000 случаев электролитных нарушений и 88 000 случаев острой почечной недостаточности[3616]. Вы видите, с какой сложной задачей сталкиваются комитеты по разработке рекомендаций!
С одной стороны, снижение артериального давления полезно для сердца, почек и мозга, но в определенный момент побочные эффекты от применения лекарств могут свести пользу на нет[3617]. В идеале мы хотим добиться как можно более низкого артериального давления у пациентов[3618], но использовать для этого лекарства можно только тогда, «когда эффект от лечения, вероятно, будет менее разрушительным, чем повышенное АД». Проблема заключается в том, что большинство людей, умирающих от сердечно-сосудистых заболеваний, сердечной недостаточности и инсульта, могут находиться в пограничном диапазоне – в зоне риска, но не настолько высоком, чтобы требовалось медикаментозное лечение[3619].
Если бы только существовал способ снизить артериальное давление без лекарств! К счастью, он есть: регулярные аэробные физические нагрузки, снижение веса, отказ от курения, увеличение потребления пищевых волокон, снижение потребления алкоголя и соли, растительная диета. Преимущества не ограничиваются отсутствием побочных эффектов. Здоровый образ жизни, в том числе растительная диета, может оказаться более эффективным, чем лекарственные препараты, поскольку вы лечите основную причину и можете наслаждаться полезными побочными эффектами[3620].
Ниже и дольше
При стандартной американской диете атеросклероз – уплотнение стенок артерий – может начаться уже в подростковом возрасте[3621]. Исследователи собрали около 3000 образцов коронарных артерий и аорт – главных артерий организма – жертв несчастных случаев, убийств и самоубийств в возрасте от 15 до 34 лет и обнаружили у подростков жировые прожилки, которые могут превратиться в атеросклеротические бляшки в 20-летнем возрасте и усугубиться в 30-летнем, прежде чем начнут убивать[3622]. У 100 % подростков в артериях образовались жировые бляшки. У них у всех была первая стадия заболевания, и у 55–65 % из тех, кому было за тридцать, эти полоски уже превратились в атеросклеротические бляшки на стенках артерий. Я понимаю: факт, что у большинства людей в возрасте около 30 лет уже есть бляшки в артериях, пугает. Другими словами, большинство из вас, вероятно, страдают сердечно-сосудистыми заболеваниями, независимо от того, знаете вы об этом или нет. Исследователи говорят следующее: «Атеросклероз начинается в молодости»[3623].
Если бы у вас был диабет, стали бы вы ждать, пока не начнете слепнуть, чтобы начать его лечить[3624]? При сердечно-сосудистых заболеваниях нельзя просто ждать, пока появятся симптомы, поскольку первый симптом может оказаться последним. У большинства американцев, умирающих от сердечно-сосудистых заболеваний, первый симптом называется «внезапной сердечной смертью»[3625].
Унция профилактики стоит гораздо больше, чем фунт лечения, потому что от смерти нет лекарства.
Как предотвратить атеросклеротическую болезнь сердца? Снижением уровня холестерина ЛПНП с помощью диеты с достаточно низким содержанием насыщенных жиров и холестерина, то есть с ограничением мяса, вредных продуктов, молочных продуктов и яиц[3626]. «Не является ли такое радикальное предложение абсолютно непрактичным?» – задается вопросом автор обзора, опубликованного в журнале Американской кардиологической ассоциации (Journal of the American Heart Association)[3627]. Для этого потребовалась бы «полная самоотдача». Но авторы обзора, ссылаясь на успех общественного здравоохранения в борьбе с курением и снижении смертности от рака легких, утверждают, что все возможно.
Какие у нас есть доказательства того, что пожизненно низкий уровень ЛПНП предотвратит сердечно-сосудистые заболевания? Существует генетическая мутация гена PCSK9, с которой повезло родиться примерно одному из пятидесяти афроамериканцев. Она обеспечивает им уровень холестерина примерно на 40 % ниже, чем у остальной популяции, в течение всей жизни[3628]. В результате у них резко снижается вероятность развития ишемической болезни сердца – на 88 %, несмотря на другие зловещие факторы риска[3629]. У большинства людей с этой мутацией уже имелись такие заболевания, как повышенное артериальное давление, избыточный вес, курение или диабет, и это свидетельствует о том, что низкий уровень холестерина ЛПНП на протяжении всей жизни значительно уменьшает риск развития ишемической болезни сердца даже при наличии множества других факторов риска.
Снижение числа кардиологических событий, таких как инфаркты или внезапная смерть, почти на 90 % происходило только при среднем уровне ЛПНП 100 мг/дл – сравните со средним показателем в 138 мг/дл у людей без мутации. С помощью лекарств или диеты можно легко достичь уровня ЛПНП даже ниже 100[3630]. Но подождите. Почему снижение уровня холестерина ЛПНП примерно на 40 мг/дл у людей со счастливой мутацией снижает вероятность заболеть ишемической болезнью сердца почти на 90 %, в то время как такое же снижение на 40 пунктов при приеме статинов уменьшает распространенность ишемической болезни сердца лишь на 20 %? Наиболее вероятный ответ – время[3631]. Чем дольше артерии находятся под воздействием повышенного уровня ЛПНП в крови, тем больше холестерина может накопиться в стенке артерии и вызывать ее воспаление[3632].
Подобно тому как воздействие табака измеряется в пачках в год – количество выкуренных сигарет, умноженное на срок, в редакционной статье Journal of the American College of Cardiology введено понятие холестериновых лет. Таким образом, принимается в расчет период, в течение которого наши артерии купаются в холестерине[3633]. Это объясняет, почему цимане, фермеры и коренные жители Боливии, о которых я упоминал ранее, практически не страдают от ишемической болезни сердца при среднем уровне ЛПНП всего около 90. Восьмидесятилетний представитель этого народа, похоже, обладает «сосудистым возрастом» 50-летнего американца[3634]. Когда речь идет о снижении уровня ЛПНП, важно не только то, насколько он низкий, но и то, как долго сохраняется на этом уровне: чем ниже и дольше, тем лучше[3635].
Если вы получили лекарственную терапию в более позднем возрасте, то для остановки прогрессирования атеросклероза вам, возможно, придется снизить уровень ЛПНП до 70 мг/дл[3636]. Но если начать лечение в раннем возрасте, то может оказаться достаточным снизить уровень ЛПНП всего лишь до 100 мг/дл, что соответствует данным по отдельным странам, согласно которым количество сердечно-сосудистых заболеваний в среднем по популяции начинает расти при превышении показателя ЛПНП сверх 100[3637]. Вот почему выбор здорового образа жизни может с 90 %-ной вероятностью избавить вас от риска сердечного приступа, в то время как лекарства могут снизить вероятность только на 20–30 %[3638]. Но эти 90 % можно получить только в том случае, если удастся всю жизнь не превышать этот показатель.
Если вы используете лекарства в конце жизни, чтобы попытаться остановить прогрессирование болезни, вам необходимо снизить уровень ЛПНП до 70 мг/дл, но для того, чтобы с помощью лекарств избавиться от последствий неправильного выбора пищи, вам, вероятно, придется снизить этот показатель примерно до 55. А если болезнь сердца настолько серьезная, что у вас уже был инфаркт и вы пытаетесь не умереть от еще одного, то в идеале вам нужно снизить уровень ЛПНП примерно до 30[3639]. При таком низком уровне вы не только предотвратите образование новых атеросклеротических бляшек[3640], но и поможете стабилизировать уже имеющиеся бляшки, чтобы они не разрывались и не убивали вас[3641].
Насколько эффективны статины?
Зачем сокращать количество продуктов, если можно просто принять таблетку? Я подробно обсуждаю эффективность статинов в ролике see.nf/statins. Абсолютное снижение риска составляет всего 1 %, поэтому на каждые 100 человек, принимающих в течение нескольких лет статины, только один избегает инфаркта[3642]. Однако большинство людей, ежедневно принимающих препарат для снижения уровня холестерина, говорят, что им нужно снижение риска, по крайней мере в 25 раз превышающее этот показатель. Если бы пациенты знали правду, если бы они знали, как слабо действуют эти препараты, почти никто не согласился бы их принимать. Исследование ожиданий пациентов, озаглавленное «Достаточно ли профилактические препараты предотвращают заболевание?», пришло к выводу, что существует «в лучшем случае недостаток обсуждения и просвещения пациентов, а в худшем – определенная дезинформация относительно преимуществ этих препаратов»[3643].
Это звучит ужасно патерналистски, но на карту поставлены сотни тысяч жизней. Просто если бы пациентам говорили правду, многие люди умерли бы. Более 30 миллионов американцев принимают статины[3644]. Даже если эти препараты спасут одного из ста, в случае массового отказа от их приема погибнут сотни тысяч людей. В статье под названием «Парадокс профилактических таблеток» делается следующий вывод: «Ирония заключается в том, что информирование пациентов о статинах приведет к увеличению тех самых исходов, которые они призваны предотвратить»[3645].
Подходят ли вам статины?
Если у вас в анамнезе есть сердечно-сосудистые заболевания или инсульт, прием статинов рекомендован. Точка. Если у вас нет известных вам сердечно-сосудистых заболеваний, то решение должно основываться на расчете вашего персонального риска, что можно легко сделать в интернете, если вы знаете показатели холестерина и артериального давления[3646]. Смотрите, например, оценку риска Американского колледжа кардиологии[3647] (see.nf/acc), профиль риска Фрамингема[3648] (see.nf/framingham) или шкалу риска Рейнольдса[3649] (see.nf/reynolds).
Я предпочитаю оценку риска Американского колледжа кардиологии, поскольку она позволяет определить риск не только на горизонте ближайших 10 лет, но и риск на протяжении всей жизни. Согласно существующим рекомендациям, если ваш десятилетний риск ниже 5 %, то для дальнейшего снижения этого показателя следует придерживаться диеты, физических упражнений и отказа от курения, если только нет противопоказаний. Если десятилетний риск достигает 20 % и выше, то к изменению образа жизни рекомендуется добавить прием статинов. В диапазоне от 5 до 7,5 % рекомендуется придерживаться нынешнего образа жизни, если у вас нет факторов, повышающих риск, а в диапазоне от 7,5 до 20 % большинство склоняется к добавлению препаратов. К факторам риска, которые врач должен учитывать при принятии решения, относятся семейная история болезней сердца или инсульта, действительно высокий уровень ЛПНП (≥ 160 мг/дл), метаболический синдром, хронические заболевания почек или воспалительные процессы, а также постоянно высокий уровень триглицеридов (≥ 175 мг/дл), С-реактивного белка (≥ 2,0 мг/л) или липопротеина (а) (≥ 50 мг/дл – см. с. 304)[3650].
Если вы все еще не уверены, стоит ли вам принимать статины, руководство Американской кардиологической ассоциации предлагает рассмотреть возможность проведения теста на определение уровня кальция в коронарных артериях[3651], хотя Рабочая группа по профилактике заболеваний США прямо заявила, что имеющихся данных недостаточно, чтобы сделать вывод о том, не перевешивает ли вред от теста пользу (даже несмотря на относительно низкую лучевую нагрузку в наши дни)[3652].
Насколько безопасны статины?
По данным исследований, до 75 % людей прекращают прием назначенных им статинов[3653]. Отвечая на вопрос о причинах, большинство из них в качестве основной причины отказа от их приема называют мышечные боли[3654]. До 72 % всех побочных эффектов статинов составляют мышечные симптомы, связанные с приемом препарата[3655]. Прием добавок с коэнзимом Q для лечения мышечных симптомов, связанных со статинами, в теории кажется хорошей идеей[3656], но на практике не работает[3657]. Обычно симптомы проходят после прекращения приема препарата, но иногда они могут сохраняться в течение года и более[3658]. Мышечные симптомы могут быть также случайными или психосоматическими и не иметь никакого отношения к препарату. Многие клинические исследования показывают, что такие побочные эффекты встречаются редко, хотя не исключено, что в этих исследованиях, финансируемых фармацевтическими компаниями, данные о них занижаются[3659].
Однако даже в ходе финансируемых фармацевтическими гигантами исследований ученые обнаружили, что вероятность развития диабета 2-го типа у принимавших эти препараты была значительно выше, чем у тех, кто принимал плацебо[3660]. Почему? Мы до сих пор точно не знаем, но, возможно, статины оказывают двойное действие: нарушают секрецию инсулина поджелудочной железой, а также снижают эффективность инсулина, повышая инсулинорезистентность[3661]. Как это ни печально, но риск сохраняется в течение многих лет даже после прекращения приема статинов[3662].
Полезны ли добавки с красным дрожжевым рисом?
Прием добавок с красным дрожжевым рисом, содержащих плесень, вырабатывающую статины, не рекомендован[3663], поскольку в них были обнаружены значительные различия в содержании активных компонентов (например, стократное различие в уровне ловастатина). Кроме того, треть проверенных добавок с красным дрожжевым рисом была загрязнена потенциально опасным для почек грибковым токсином цитринином[3664]. Обновленный анализ 2021 года выявил превышение уровня безопасности цитринина в 97 % отобранных добавок, включая добавки с маркировкой «без цитринина», что представляет собой «серьезную проблему для здоровья»[3665].
Учитывая роль статинов в снижении частоты сердечно-сосудистых заболеваний, являющихся главной причиной смерти, любое увеличение риска развития диабета, который обычно является седьмой причиной смерти (восьмой – с учетом COVID)[3666], будет менее значимым в сравнении с преимуществами в отношении риска сердечно-сосудистых заболеваний[3667]. Ожидается, что у лиц, принимающих статины, будут развиваться дополнительно два случая сахарного диабета на тысячу пациенто-лет, и за это время будет предотвращено шесть с половиной сердечно-сосудистых событий, таких как инфаркты или инсульты[3668]. Конечно, это ложная дихотомия[3669]. Нам не нужно выбирать между сердечно-сосудистыми заболеваниями и диабетом. Мы можем лечить причины обоих заболеваний с помощью одной и той же диеты и изменения образа жизни. Диета, которая не только останавливает прогрессирование сердечно-сосудистых заболеваний, но и обращает их вспять[3670], – это тот же самый способ питания, который позволяет добиться ремиссии диабета 2-го типа[3671]. Здоровая растительная диета может предотвратить дальнейшие тяжелые сердечные приступы у 99,4 % пациентов с серьезными заболеваниями сердца[3672].
Что насчет ингибиторов PCSK9?
Экстраполяция данных, полученных в ходе крупных исследований по снижению уровня холестерина, позволяет предположить, что частота сердечно-сосудистых событий, таких как инфаркты, будет приближаться к нулю, если уровень холестерина ЛПНП удастся снизить до 60 мг/дл у лиц, никогда не имевших инфаркта, и до 30 мг/дл у тех, кто пытается предотвратить его повторение[3673]. Безопасно ли вообще иметь такой низкий уровень холестерина? До открытия ингибиторов PCSK9 мы этого не знали[3674].
Если вы помните, PCSK9 – это мутация гена, благодаря которой у некоторых людей пожизненно низкий уровень ЛПНП[3675]. Компании, производящие лекарственные препараты, были вдохновлены природной мутацией и решили изобрести фармакологический способ воздействия на этот ген[3676]. Полное описание смотрите в ролике see.nf/pcsk9. Принимая ингибиторы PCSK9, люди могут достичь уровня ЛПНП ниже 40 мг/дл, а некоторые даже ниже 15 мг/дл[3677]. Риск сердечных приступов снижается пропорционально снижению уровня ЛПНП, вплоть до уровня ниже 10 мг/дл без видимых проблем с безопасностью, таких как нарушение синтеза гормонов надпочечников, яичников или яичек, которые организм вырабатывает из холестерина[3678].
Люди с экстремальными мутациями PCSK9, которые приводят к снижению уровня ЛПНП до уровня менее 20 мг/дл на протяжении всей жизни, остаются здоровыми и рожают здоровых детей[3679]. Существует и другой тип генетических мутаций, при которых уровень ЛПНП в течение всей жизни составляет около 30 мг/дл, и такие люди отличаются исключительно высокой продолжительностью жизни[3680]. Мутации, влияющие на уровень холестерина, фактически являются причиной так называемых синдромов долголетия, но это не означает, что лекарства безопасны[3681]. В итоге можно сказать, что мы должны стараться снизить уровень холестерина ЛПНП настолько, насколько это возможно, однако при появлении нового класса препаратов необходимы более длительные наблюдения[3682]. Пока все хорошо, но прошло всего несколько лет. Например, мы узнали о том, что статины повышают риск развития диабета, только через 10 лет после того, как они были одобрены, и миллионы людей уже пострадали от них[3683]. Следует также отметить, что стоимость курса ингибиторов PCSK9 составляет около 14 000 долларов в год[3684].
Великая афера со стентами
Помимо личных привычек и предубеждений, пренебрежительное отношение к образу жизни формируется у пациентов как реакция на увлеченность кардиологов всеми модными гаджетами и новыми процедурами[3685]. Некоторые из них чувствуют, что их обучали как высококвалифицированных летчиков-истребителей, готовых вступить в бой с применением высокотехнологичного оружия, а теперь вынуждают отправиться в скучную профилактическую дипломатическую миссию. Мало того что упускается возможность лечения и устранения основной причины заболевания, некоторые распространенные кардиологические методы, как показывает практика, приносят больше вреда, чем пользы. Я не призываю придираться к кардиологам, но многие современные медицинские практики, как выяснилось, потенциально опасны[3686]. По оценкам самих врачей, примерно пятая часть медицинской помощи является ненужной[3687].
Моя серия из семи видеороликов о стентах и ангиопластике начинается с see.nf/stents. Итог: при инфаркте установка стентов может спасти жизнь, но сотни тысяч таких процедур проводятся при стабильной стенокардии, то есть не в экстренных случаях[3688]. Считалось, что они смягчают симптомы[3689], но на самом деле они не продлевают жизнь и не снижают риск возникновения инфаркта в будущем в сравнении с медикаментозной терапией, которая подразумевает коррекцию образа жизни и прием статинов[3690]. Как говорится в Harvard Heart Letter, «стенты – это от боли, а не для защиты»[3691]. Но затем в ходе двойного слепого рандомизированного контролируемого исследования[3692] было обнаружено, что стенты, возможно, не помогают и от боли.
Подождите, как так? Двойное слепое, рандомизированное, контролируемое исследование, включающее хирургическое вмешательство? При испытании лекарств можно дать участникам исследования сахарную таблетку-плацебо, чтобы они не знали, находятся они в группе активного лечения или в контрольной группе, но разве вы не заметите, если кто-то разрежет вам пах? Нет, если вам сделали мнимую операцию[3693]. Да, плацебо-хирургия – это что-то. В ходе исследования ученые разрезали пах каждого пациента, вводили катетер, а затем устанавливали или не устанавливали настоящий стент. И те, кому была проведена мнимая операция, испытывали такое же облегчение, как и те, кому была проведена настоящая операция[3694].
Если причиной инфаркта является закупорка артерий, то почему физическое вскрытие артерий не помогает? Большинство инфарктов вызывается сужениями, блокирующими менее 70 % артерий, поэтому бляшки-убийцы не видны на ангиограммах[3695]. До разрыва эти бляшки часто не ограничивают кровоток, поэтому они могут быть незаметны при ангиографии и нагрузочных тестах[3696]. Поэтому наиболее опасные поражения могут не поддаваться ангиопластике и стентированию, которые никак не меняют течения основного заболевания.
Устранение причины
Чтобы резко снизить уровень холестерина ЛПНП, необходимо решительно сократить потребление трех компонентов, повышающих его: трансжиров, насыщенных жиров и пищевого холестерина[3697]. В США трансжиры, содержащие частично гидрогенизированные масла, некогда были распространенным ингредиентом ультрапереработанных пищевых продуктов, а затем были фактически запрещены в этой стране и ограничены в десятках других стран мира[3698]. В настоящее время в странах, где проводилось исследование, выяснили, что основная часть трансжиров поступает в организм из мяса и молочных продуктов[3699]. Насыщенные жиры, повышающие уровень холестерина, содержатся в основном в продуктах животного происхождения и «мусорной» пище. В США основным источником насыщенных жиров являются молочные продукты, за ними следует курица, затем выпечка (включая пиццу), свинина и гамбургеры[3700]. Пищевой холестерин содержится исключительно в продуктах животного происхождения[3701], и его источник номер один – яйца. Курица занимает вторую позицию, за ней следуют говядина, молочные продукты и свинина[3702]. Поэтому неудивительно, что основная диетическая рекомендация ведущих научных обществ кардиологов по профилактике сердечно-сосудистых заболеваний заключается в том, чтобы «делать упор на потребление продуктов растительного, а не животного происхождения»[3703].
Рандомизированные контролируемые исследования с участием более 50 тысяч человек показали, что сокращение потребления насыщенных жиров приводит к снижению частоты сердечно-сосудистых заболеваний, причем чем больше мы уменьшаем содержание насыщенных жиров, тем значительнее снижается уровень холестерина. В Cochrane Review[3704], считающемся золотым стандартом исследований, делается следующее заключение: «В группах населения с пониженным риском следует продолжать постоянное снижение содержания насыщенных жиров в пище»[3705]. (Арчи Кокрейн был пионером доказательной медицины, чье наследие увековечено в названии некоммерческой организации, уважаемой за высококачественные систематические обзоры). Американская кардиологическая ассоциация настолько устала от попыток производителей сливочного масла убедить людей в том, что сливочное масло не вредно, что выпустила консультативный документ[3706], «чтобы прояснить, почему хорошо проведенные научные исследования в подавляющем большинстве случаев поддерживают ограничение насыщенных жиров в рационе»[3707].
Запрет на употребление насыщенных жиров распространяется и на тропические масла, которые часто используются в нездоровой пище, в том числе кокосовое, пальмовое и пальмоядровое[3708], хотя животные источники, по-видимому, хуже. В исследовании APPROACH (Animal and Plant PROtein And Cardiovascular Health – Животные и растительные белки и здоровье сердечно-сосудистой системы) люди были рандомизированы на три группы: придерживающихся 1) диеты с высоким содержанием насыщенных жиров, состоящие из источников белка из красного мяса; 2) с низким содержанием насыщенных жиров – белого мяса или 3) вообще без мяса (бобовые, зерновые, орехи). Исследователи скорректировали рацион таким образом, чтобы добиться одинакового потребления насыщенных жиров во всех трех группах, используя сливочное масло в двух «мясных» группах и тропические масла в группе немясной диеты. Результаты? При одинаковом потреблении насыщенных жиров и красное и белое мясо повышали уровень холестерина ЛПНП больше, чем растительные источники белка[3709]. Оказалось, что красное и белое мясо одинаково вредно, что характерно даже для рандомизированных контролируемых исследований, в которых не нормировался уровень насыщенных жиров. Замена говядины на курицу и/или рыбу не приводит к значительному снижению уровня холестерина ЛПНП[3710].