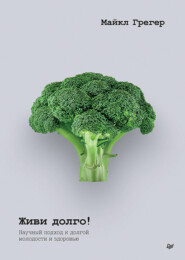
Полная версия:
Живи долго! Научный подход к долгой молодости и здоровью
1645
Leenen R, Roodenburg AJ, Tijburg LB, Wiseman SA. A single dose of tea with or without milk increases plasma antioxidant activity in humans. Eur J Clin Nutr. 2000;54(1):87–92. https://pubmed.ncbi.nlm.nih.gov/10694777/
1646
Rashidinejad A, Birch EJ, Sun-Waterhouse D, Everett DW. Addition of milk to tea infusions: helpful or harmful? Evidence from in vitro and in vivo studies on antioxidant properties. Crit Rev Food Sci Nutr. 2017;57(15):3188–96. https://pubmed.ncbi.nlm.nih.gov/26517348/
1647
Ho CK, Choi SW, Siu PM, Benzie IFF. Effects of single dose and regular intake of green tea (Camellia sinensis) on DNA damage, DNA repair, and heme oxygenase-1 expression in a randomized controlled human supplementation study. Mol Nutr Food Res. 2014;58(6):1379–83. https://pubmed.ncbi.nlm.nih.gov/24585444/
1648
Han KC, Wong WC, Benzie IFF. Genoprotective effects of green tea (Camellia sinensis) in human subjects: results of a controlled supplementation trial. Br J Nutr. 2011;105(2):171–9. https://pubmed.ncbi.nlm.nih.gov/20807462/
1649
Dias TR, Alves MG, Tomás GD, Socorro S, Silva BM, Oliveira PF. White tea as a promising antioxidant medium additive for sperm storage at room temperature: a comparative study with green tea. J Agric Food Chem. 2014;62(3):608–17. https://pubmed.ncbi.nlm.nih.gov/24372402/
1650
Choi SW, Yeung VTF, Collins AR, Benzie IFF. Redox-linked effects of green tea on DNA damage and repair, and influence of microsatellite polymorphism in HMOX-1: results of a human intervention trial. Mutagenesis. 2015;30(1):129–37. https://pubmed.ncbi.nlm.nih.gov/25527735/
1651
Leaf DA, Kleinman MT, Hamilton M, Deitrick RW. The exercise-induced oxidative stress paradox: the effects of physical exercise training. Am J Med Sci. 1999;317(5):295–300. https://pubmed.ncbi.nlm.nih.gov/10334116/
1652
Mastaloudis A, Yu TW, O’Donnell RP, Frei B, Dashwood RH, Traber MG. Endurance exercise results in DNA damage as detected by the comet assay. Free Radic Biol Med. 2004;36(8):966–75. https://pubmed.ncbi.nlm.nih.gov/15059637/
1653
Vollaard NBJ, Shearman JP, Cooper CE. Exercise-induced oxidative stress: myths, realities and physiological relevance. Sports Med. 2005;35(12):1045–62. https://pubmed.ncbi.nlm.nih.gov/16336008/
1654
Mastaloudis A, Yu TW, O’Donnell RP, Frei B, Dashwood RH, Traber MG. Endurance exercise results in DNA damage as detected by the comet assay. Free Radic Biol Med. 2004;36(8):966–75. https://pubmed.ncbi.nlm.nih.gov/15059637/
1655
Fisher-Wellman K, Bloomer RJ. Acute exercise and oxidative stress: a 30 year history. Dyn Med. 2009;8:1. https://pubmed.ncbi.nlm.nih.gov/19144121/
1656
Ristow M, Zarse K, Oberbach A, et al. Antioxidants prevent health-promoting effects of physical exercise in humans. Proc Natl Acad Sci U S A. 2009;106(21):8665–70. https://pubmed.ncbi.nlm.nih.gov/19433800/
1657
Braakhuis AJ. Effect of vitamin C supplements on physical performance. Curr Sports Med Rep. 2012;11(4):180–4. https://pubmed.ncbi.nlm.nih.gov/22777327/
1658
Kashi DS, Shabir A, Da Boit M, Bailey SJ, Higgins MF. The efficacy of administering fruit-derived polyphenols to improve health biomarkers, exercise performance and related physiological responses. Nutrients. 2019;11(10):E2389. https://pubmed.ncbi.nlm.nih.gov/31591287/
1659
Van der Avoort CMT, Van Loon LJC, Hopman MTE, Verdijk LB. Increasing vegetable intake to obtain the health promoting and ergogenic effects of dietary nitrate. Eur J Clin Nutr. 2018;72(11):1485–9. https://pubmed.ncbi.nlm.nih.gov/29559721/
1660
Trapp D, Knez W, Sinclair W. Could a vegetarian diet reduce exercise-induced oxidative stress? A review of the literature. J Sports Sci. 2010;28(12):1261–8. https://pubmed.ncbi.nlm.nih.gov/20845212/
1661
Lyall KA, Hurst SM, Cooney J, et al. Short-term blackcurrant extract consumption modulates exercise-induced oxidative stress and lipopolysaccharide-stimulated inflammatory responses. Am J Physiol Regul Integr Comp Physiol. 2009;297(1):R70–81. https://pubmed.ncbi.nlm.nih.gov/19403859/
1662
Funes L, Carrera-Quintanar L, Cerdán-Calero M, et al. Effect of lemon verbena supplementation on muscular damage markers, proinflammatory cytokines release and neutrophils’ oxidative stress in chronic exercise. Eur J Appl Physiol. 2011;111(4):695–705. https://pubmed.ncbi.nlm.nih.gov/20967458/
1663
Ghezzi P, Jaquet V, Marcucci F, Schmidt HHHW. The oxidative stress theory of disease: levels of evidence and epistemological aspects. Br J Pharmacol. 2017;174(12):1784–96. https://pubmed.ncbi.nlm.nih.gov/27425643/
1664
Scudellari M. The science myths that will not die. Nature. 2015;528(7582):322–5. https://pubmed.ncbi.nlm.nih.gov/26672537/
1665
Peng C, Wang X, Chen J, et al. Biology of ageing and role of dietary antioxidants. Biomed Res Int. 2014;2014:831841. https://pubmed.ncbi.nlm.nih.gov/24804252/
1666
Milisav I, Ribaric S, Poljsak B. Antioxidant vitamins and ageing. Subcell Biochem. 2018;90:1–23. https://pubmed.ncbi.nlm.nih.gov/30779004/
1667
Smejkal GB, Kakumanu S. Enzymes and their turnover numbers. Expert Rev Proteom. 2019;16(7):543–4. https://pubmed.ncbi.nlm.nih.gov/31220960/
1668
Raghunath A, Sundarraj K, Nagarajan R, et al. Antioxidant response elements: discovery, classes, regulation and potential applications. Redox Biol. 2018;17:297–314. https://pubmed.ncbi.nlm.nih.gov/29775961/
1669
Zang H, Mathew RO, Cui T. The dark side of Nrf2 in the heart. Front Physiol. 2020;11:722. https://pubmed.ncbi.nlm.nih.gov/32733266/
1670
Brandes MS, Gray NE. NRF2 as a therapeutic target in neurodegenerative diseases. ASN Neuro. 2020;12:1759091419899782. https://pubmed.ncbi.nlm.nih.gov/31964153/
1671
Sharma V, Kaur A, Singh TG. Counteracting role of nuclear factor erythroid 2-related factor 2 pathway in Alzheimer’s disease. Biomed Pharmacother. 2020;129:110373. https://pubmed.ncbi.nlm.nih.gov/32603894/
1672
Yuan H, Xu Y, Luo Y, Wang NX, Xiao JH. Role of Nrf2 in cell senescence regulation. Mol Cell Biochem. 2021;476(1):247–59. https://pubmed.ncbi.nlm.nih.gov/32918185/
1673
Raghunath A, Sundarraj K, Nagarajan R, et al. Antioxidant response elements: discovery, classes, regulation and potential applications. Redox Biol. 2018;17:297–314. https://pubmed.ncbi.nlm.nih.gov/29775961/
1674
Raghunath A, Sundarraj K, Nagarajan R, et al. Antioxidant response elements: discovery, classes, regulation and potential applications. Redox Biol. 2018;17:297–314. https://pubmed.ncbi.nlm.nih.gov/29775961/
1675
Ferguson LR, Schlothauer RC. The potential role of nutritional genomics tools in validating high health foods for cancer control: broccoli as example. Mol Nutr Food Res. 2012;56(1):126–46. https://pubmed.ncbi.nlm.nih.gov/22147677/
1676
Sun Y, Yang T, Leak RK, Chen J, Zhang F. Preventive and protective roles of dietary Nrf2 activators against central nervous system diseases. CNS Neurol Disord Drug Targets. 2017;16(3):326–38. https://pubmed.ncbi.nlm.nih.gov/28042770/
1677
Yang L, Palliyaguru DL, Kensler TW. Frugal chemoprevention: targeting Nrf2 with foods rich in sulforaphane. Semin Oncol. 2016;43(1):146–53. https://pubmed.ncbi.nlm.nih.gov/26970133/
1678
Qu Z, Sun J, Zhang W, Yu J, Zhuang C. Transcription factor NRF2 as a promising therapeutic target for Alzheimer’s disease. Free Radic Biol Med. 2020;159:87–102. https://pubmed.ncbi.nlm.nih.gov/32730855/
1679
Lewis KN, Mele J, Hayes JD, Buffenstein R. Nrf2, a guardian of healthspan and gatekeeper of species longevity. Integr Comp Biol. 2010;50(5):829–43. https://pubmed.ncbi.nlm.nih.gov/21031035/
1680
Tullet JMA, Hertweck M, An JH, et al. Direct inhibition of the longevity-promoting factor SKN-1 by insulin-like signaling in C. elegans. Cell. 2008;132(6):1025–38. https://pubmed.ncbi.nlm.nih.gov/18358814/
1681
Sykiotis GP, Bohmann D. Keap1/Nrf2 signaling regulates oxidative stress tolerance and lifespan in Drosophila. Dev Cell. 2008;14(1):76–85. https://pubmed.ncbi.nlm.nih.gov/18194654/
1682
Lewis KN, Wason E, Edrey YH, Kristan DM, Nevo E, Buffenstein R. Regulation of Nrf2 signaling and longevity in naturally long-lived rodents. Proc Natl Acad Sci U S A. 2015;112(12):3722–7. https://pubmed.ncbi.nlm.nih.gov/25775529/
1683
Yu C, Li Y, Holmes A, et al. RNA sequencing reveals differential expression of mitochondrial and oxidation reduction genes in the long-lived naked mole-rat when compared to mice. PLoS ONE. 2011;6(11):e26729. https://pubmed.ncbi.nlm.nih.gov/22073188/
1684
Lewis KN, Wason E, Edrey YH, Kristan DM, Nevo E, Buffenstein R. Regulation of Nrf2 signaling and longevity in naturally long-lived rodents. Proc Natl Acad Sci U S A. 2015;112(12):3722–7. https://pubmed.ncbi.nlm.nih.gov/25775529/
1685
Andziak B, O’Connor TP, Buffenstein R. Antioxidants do not explain the disparate longevity between mice and the longest-living rodent, the naked mole-rat. Mech Ageing Dev. 2005;126(11):1206–12. https://pubmed.ncbi.nlm.nih.gov/16087218/
1686
Lewis KN, Wason E, Edrey YH, Kristan DM, Nevo E, Buffenstein R. Regulation of Nrf2 signaling and longevity in naturally long-lived rodents. Proc Natl Acad Sci U S A. 2015;112(12):3722–7. https://pubmed.ncbi.nlm.nih.gov/25775529/
1687
Yuan H, Xu Y, Luo Y, Wang NX, Xiao JH. Role of Nrf2 in cell senescence regulation. Mol Cell Biochem. 2021;476(1):247–59. https://pubmed.ncbi.nlm.nih.gov/32918185/
1688
Zhou L, Zhang H, Davies KJA, Forman HJ. Aging-related decline in the induction of Nrf2-regulated antioxidant genes in human bronchial epithelial cells. Redox Biol. 2018;14:35–40. https://pubmed.ncbi.nlm.nih.gov/28863281/
1689
Mallard AR, Spathis JG, Coombes JS. Nuclear factor (erythroid-derived 2)-like 2 (Nrf2) and exercise. Free Radic Biol Med. 2020;160:471–9. https://pubmed.ncbi.nlm.nih.gov/32871230/
1690
Zhang DD, Chapman E. The role of natural products in revealing NRF2 function. Nat Prod Rep. 2020;37(6):797–826. https://pubmed.ncbi.nlm.nih.gov/32400766/
1691
Su X, Jiang X, Meng L, Dong X, Shen Y, Xin Y. Anticancer activity of sulforaphane: the epigenetic mechanisms and the Nrf2 signaling pathway. Oxid Med Cell Longev. 2018;2018:5438179. https://pubmed.ncbi.nlm.nih.gov/29977456/
1692
Bose C, Alves I, Singh P, et al. Sulforaphane prevents age-associated cardiac and muscular dysfunction through Nrf2 signaling. Aging Cell. 2020;19(11):e13261. https://pubmed.ncbi.nlm.nih.gov/33067900/
1693
Kubo E, Chhunchha B, Singh P, Sasaki H, Singh DP. Sulforaphane reactivates cellular antioxidant defense by inducing Nrf2/ARE/Prdx6 activity during aging and oxidative stress. Sci Rep. 2017;7:14130. https://pubmed.ncbi.nlm.nih.gov/29074861/
1694
Yuan H, Xu Y, Luo Y, Wang NX, Xiao JH. Role of Nrf2 in cell senescence regulation. Mol Cell Biochem. 2021;476(1):247–59. https://pubmed.ncbi.nlm.nih.gov/32918185/
1695
Riso P, Martini D, Møller P, et al. DNA damage and repair activity after broccoli intake in young healthy smokers. Mutagenesis. 2010;25(6):595–602. https://pubmed.ncbi.nlm.nih.gov/20713433/
1696
Hoelzl C, Glatt H, Meinl W, et al. Consumption of Brussels sprouts protects peripheral human lymphocytes against 2-amino-1-methyl-6-phenylimidazo[4,5-b]pyridine (PhIP) and oxidative DNA-damage: results of a controlled human intervention trial. Mol Nutr Food Res. 2008;52(3):330–41. https://pubmed.ncbi.nlm.nih.gov/18293303/
1697
Egner PA, Chen JG, Zarth AT, et al. Rapid and sustainable detoxication of airborne pollutants by broccoli sprout beverage: results of a randomized clinical trial in China. Cancer Prev Res. 2014;7(8):813–23. https://pubmed.ncbi.nlm.nih.gov/24913818/
1698
Heber D, Li Z, Garcia-Lloret M, et al. Sulforaphane-rich broccoli sprout extract attenuates nasal allergic response to diesel exhaust particles. Food Funct. 2014;5(1):35–41. https://pubmed.ncbi.nlm.nih.gov/24287881/
1699
Eagles SK, Gross AS, McLachlan AJ. The effects of cruciferous vegetable-enriched diets on drug metabolism: a systematic review and meta-analysis of dietary intervention trials in humans. Clin Pharmacol Ther. 2020;108(2):212–27. https://pubmed.ncbi.nlm.nih.gov/32086800/
1700
Knatko EV, Ibbotson SH, Zhang Y, et al. Nrf2 activation protects against solar-simulated ultraviolet radiation in mice and humans. Cancer Prev Res (Phila). 2015;8(6):475–86. https://pubmed.ncbi.nlm.nih.gov/25804610/
1701
Houghton CA, Fassett RG, Coombes JS. Sulforaphane and other nutrigenomic Nrf2 activators: can the clinician’s expectation be matched by the reality? Oxid Med Cell Longev. 2016;2016:7857186. https://pubmed.ncbi.nlm.nih.gov/26881038/
1702
Aune D, Giovannucci E, Boffetta P, et al. Fruit and vegetable intake and the risk of cardiovascular disease, total cancer and all-cause mortality – a systematic review and dose-response meta-analysis of prospective studies. Int J Epidemiol. 2017;46(3):1029–56. https://pubmed.ncbi.nlm.nih.gov/28338764/
1703
Mori N, Shimazu T, Charvat H, et al. Cruciferous vegetable intake and mortality in middle-aged adults: a prospective cohort study. Clin Nutr. 2019;38(2):631–43. https://pubmed.ncbi.nlm.nih.gov/29739681/
1704
Grünwald S, Stellzig J, Adam IV, et al. Longevity in the red flour beetle Tribolium castaneum is enhanced by broccoli and depends on nrf-2, jnk-1 and foxo-1 homologous genes. Genes Nutr. 2013;8(5):439–48. https://pubmed.ncbi.nlm.nih.gov/23321956/
1705
Hanschen FS. Domestic boiling and salad preparation habits affect glucosinolate degradation in red cabbage (Brassica oleracea var. capitata f. rubra). Food Chem. 2020;321:126694. https://pubmed.ncbi.nlm.nih.gov/32244140/
1706
Hernández-Ruiz Á, García-Villanova B, Guerra-Hernández E, Amiano P, Ruiz-Canela M, Molina-Montes E. A review of a priori defined oxidative balance scores relative to their components and impact on health outcomes. Nutrients. 2019;11(4):774. https://pubmed.ncbi.nlm.nih.gov/30987200/
1707
Holland RD, Gehring T, Taylor J, Lake BG, Gooderham NJ, Turesky RJ. Formation of a mutagenic heterocyclic aromatic amine from creatinine in urine of meat eaters and vegetarians. Chem Res Toxicol. 2005;18(3):579–90. https://pubmed.ncbi.nlm.nih.gov/15777097/
1708
Carvalho AM, Miranda AM, Santos FA, Loureiro APM, Fisberg RM, Marchioni DM. High intake of heterocyclic amines from meat is associated with oxidative stress. Br J Nutr. 2015;113(8):1301–7. https://pubmed.ncbi.nlm.nih.gov/25812604/
1709
Macho-González A, Garcimartín A, López-Oliva ME, et al. Can meat and meat-products induce oxidative stress? Antioxidants (Basel). 2020;9(7):638. https://pubmed.ncbi.nlm.nih.gov/32698505/
1710
Kanner J, Lapidot T. The stomach as a bioreactor: dietary lipid peroxidation in the gastric fluid and the effects of plant-derived antioxidants. Free Radic Biol Med. 2001;31(11):1388–95. https://pubmed.ncbi.nlm.nih.gov/11728810/
1711
Mohamed B, Mohamed I. The effects of residual blood of carcasses on poultry technological quality. Food Nutri Sci. 2012;03(10):1382–6. https://www.scirp.org/journal/paperinformation.aspx?paperid=23386
1712
Alvarado CZ, Richards MP, O’Keefe SF, Wang H. The effect of blood removal on oxidation and shelf life of broiler breast meat. Poult Sci. 2007;86(1):156–61. https://pubmed.ncbi.nlm.nih.gov/17179431/
1713
Cohn JS. Oxidized fat in the diet, postprandial lipaemia and cardiovascular disease. Curr Opin Lipidol. 2002;13(1):19–24. https://pubmed.ncbi.nlm.nih.gov/11790959/
1714
Gorelik S, Kanner J, Schurr D, Kohen R. A rational approach to prevent postprandial modification of LDL by dietary polyphenols. J Funct Foods. 2013;5(1):163–9. https://www.sciencedirect.com/science/article/pii/S1756464612001466?via%3Dihub
1715
Jafari S, Hezaveh E, Jalilpiran Y, et al. Plant-based diets and risk of disease mortality: a systematic review and meta-analysis of cohort studies. Crit Rev Food Sci Nutr. https://www.tandfonline.com/doi/full/10.1080/10408398.2021.1918628. Published May 6, 2021. Accessed July 10, 2021.; https://www.tandfonline.com/doi/full/10.1080/10408398.2021.1918628
1716
Cohn JS. Oxidized fat in the diet, postprandial lipaemia and cardiovascular disease. Curr Opin Lipidol. 2002;13(1):19–24. https://pubmed.ncbi.nlm.nih.gov/11790959/
1717
Edalati S, Bagherzadeh F, Asghari Jafarabadi M, Ebrahimi-Mamaghani M. Higher ultra-processed food intake is associated with higher DNA damage in healthy adolescents. Br J Nutr. 2021;125(5):568–76. https://pubmed.ncbi.nlm.nih.gov/32513316/
1718
Macho-González A, Garcimartín A, López-Oliva ME, et al. Can meat and meat-products induce oxidative stress? Antioxidants (Basel). 2020;9(7):638. https://pubmed.ncbi.nlm.nih.gov/32698505/
1719
Aleksandrova K, Koelman L, Rodrigues CE. Dietary patterns and biomarkers of oxidative stress and inflammation: a systematic review of observational and intervention studies. Redox Biol. 2021;42:101869. https://pubmed.ncbi.nlm.nih.gov/33541846/
1720
Benzie IFF, Wachtel-Galor S. Vegetarian diets and public health: biomarker and redox connections. Antioxid Redox Signal. 2010;13(10):1575–91. https://pubmed.ncbi.nlm.nih.gov/20222825/
1721
Burri BJ. Antioxidant status in vegetarians versus omnivores: a mechanism for longer life? Nutrition. 2000;16(2):149–50. https://pubmed.ncbi.nlm.nih.gov/10755825/
1722
Krajcovicová-Kudlácková M, Šimoncic R, Béderová A, Klvanová J, Brtková A, Grancicová E. Lipid and antioxidant blood levels in vegetarians. Nahrung. 1996;40(1):17–20. https://pubmed.ncbi.nlm.nih.gov/8975140/
1723
Kováciková Z, Cerhata D, Kadrabová J, Madaric A, Ginter E. Antioxidant status in vegetarians and nonvegetarians in Bratislava region (Slovakia). Z Ernahrungswiss. 1998;37(2):178–82. https://pubmed.ncbi.nlm.nih.gov/9698645/
1724
Nagyová A, Kudlácková M, Grancicová E, Magálová T. LDL oxidizability and antioxidative status of plasma in vegetarians. Ann Nutr Metab. 1998;42(6):328–32. https://pubmed.ncbi.nlm.nih.gov/9895420/
1725
Boanca MM, Colosi HA, Craciun EC. The impact of the lacto-ovo vegetarian diet on the erythrocyte superoxide dismutase activity: a study in the Romanian population. Eur J Clin Nutr. 2014;68(2):184–8. https://pubmed.ncbi.nlm.nih.gov/24105324/
1726
Krajcovicová-Kudlácková M, Valachovicová M, Pauková V, Dušinská M. Effects of diet and age on oxidative damage products in healthy subjects. Physiol Res. 2008;57(4):647–51. https://pubmed.ncbi.nlm.nih.gov/17705666/
1727
Somannavar MS, Kodliwadmath MV. Correlation between oxidative stress and antioxidant defence in South Indian urban vegetarians and non-vegetarians. Eur Rev Med Pharmacol Sci. 2012;16(3):351–4. https://pubmed.ncbi.nlm.nih.gov/22530352/
1728
Manjari V, Suresh Y, Sailaja Devi MM, Das UN. Oxidant stress, anti-oxidants and essential fatty acids in South Indian vegetarians and non-vegetarians. Prostaglandins Leukot Essent Fatty Acids. 2001;64(1):53–9. https://pubmed.ncbi.nlm.nih.gov/11161585/
1729
Kim MK, Cho SW, Park YK. Long-term vegetarians have low oxidative stress, body fat, and cholesterol levels. Nutr Res Pract. 2012;6(2):155–61. https://pubmed.ncbi.nlm.nih.gov/22586505/
1730
Szeto YT, Kwok TCY, Benzie IFF. Effects of a long-term vegetarian diet on biomarkers of antioxidant status and cardiovascular disease risk. Nutrition. 2004;20(10):863–6. https://pubmed.ncbi.nlm.nih.gov/15474873/
1731
Gajski G, Geric M, Vucic Lovrencic M, et al. Analysis of health-related biomarkers between vegetarians and non-vegetarians: a multi-biomarker approach. J Funct Foods. 2018;48:643–53. https://www.sciencedirect.com/science/article/abs/pii/S1756464618304109?via%3Dihub
1732
Poornima K, Cariappa M, Asha K, Kedilaya HP, Nandini M. Oxidant and antioxidant status in vegetarians and fish eaters. Indian J Clin Biochem. 2003;18(2):197–205. https://pubmed.ncbi.nlm.nih.gov/23105412/
1733
Krajcovicová-Kudlácková M, Šimoncic R, Babinská K, Béderová A. Levels of lipid peroxidation and antioxidants in vegetarians. Eur J Epidemiol. 1995;11(2):207–11. https://pubmed.ncbi.nlm.nih.gov/7672077/
1734
Nadimi H, Yousefinejad A, Djazayery A, Hosseini M, Hosseini S. Association of vegan diet with RMR, body composition and oxidative stress. Acta Sci Pol Technol Aliment. 2013;12(3):311–8. https://pubmed.ncbi.nlm.nih.gov/24584960/
1735
Herrmann W, Schorr H, Purschwitz K, Rassoul F, Richter V. Total homocysteine, vitamin B12, and total antioxidant status in vegetarians. Clin Chem. 2001;47(6):1094–101. https://pubmed.ncbi.nlm.nih.gov/11375297/
1736
van de Lagemaat EE, de Groot LCPGM, van den Heuvel EGHM. Vitamin B12 in relation to oxidative stress: a systematic review. Nutrients. 2019;11(2):E482. https://pubmed.ncbi.nlm.nih.gov/30823595/
1737
Pawlak R, Lester SE, Babatunde T. The prevalence of cobalamin deficiency among vegetarians assessed by serum vitamin B12: a review of literature. Eur J Clin Nutr. 2014;68(5):541–8. https://pubmed.ncbi.nlm.nih.gov/24667752/
1738
Poli G, Biasi F, Leonarduzzi G. Oxysterols in the pathogenesis of major chronic diseases. Redox Biol. 2013;1:125–30. https://pubmed.ncbi.nlm.nih.gov/24024145/
1739
Wellington CL, Frikke-Schmidt R. Relation between plasma and brain lipids. Curr Opin Lipidol. 2016;27(3):225–32. https://pubmed.ncbi.nlm.nih.gov/27149391/
1740
Poli G, Biasi F, Leonarduzzi G. Oxysterols in the pathogenesis of major chronic diseases. Redox Biol. 2013;1:125–30. https://pubmed.ncbi.nlm.nih.gov/24024145/
1741
Gamba P, Testa G, Gargiulo S, Staurenghi E, Poli G, Leonarduzzi G. Oxidized cholesterol as the driving force behind the development of Alzheimer’s disease. Front Aging Neurosci. 2015;7. https://pubmed.ncbi.nlm.nih.gov/26150787/
1742
Otaegui-Arrazola A, Menéndez-Carreño M, Ansorena D, Astiasarán I. Oxysterols: a world to explore. Food Chem Toxicol. 2010;48(12):3289–303. https://pubmed.ncbi.nlm.nih.gov/20870006/
1743
Iuliano L, Micheletta F, Natoli S, et al. Measurement of oxysterols and a-tocopherol in plasma and tissue samples as indices of oxidant stress status. Anal Biochem. 2003;312(2):217–23. https://pubmed.ncbi.nlm.nih.gov/12531208/
1744
Zarrouk A, Vejux A, Mackrill J, et al. Involvement of oxysterols in age-related diseases and ageing processes. Ageing Res Rev. 2014;18:148–62. https://pubmed.ncbi.nlm.nih.gov/25305550/
1745