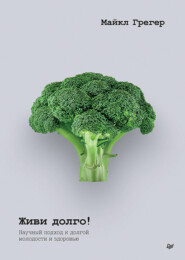
Полная версия:
Живи долго! Научный подход к долгой молодости и здоровью
Жители Окинавы традиционно употребляют в пищу вид имбиря, называемый «панцирный имбирь»[2696]. Было показано, что экстракты листьев панцирного имбиря увеличивают продолжительность жизни C. elegans, но кто ест листья имбиря[2697]? Формально мы едим не корень, а корневище имбиря, которое является подземной частью стебля[2698]. К счастью, 6-шогаол, содержащийся в обычном молотом имбире, сам по себе может увеличить среднюю продолжительность жизни C. elegans более чем на 25 %[2699].
Увеличение продолжительности жизни может быть связано с защитой ДНК. Если взять образец ткани у человека, то примерно в 7 % клеток можно обнаружить повреждения ДНК, то есть разрывы в ее нитях. Если затем подвергнуть эти клетки воздействию свободных радикалов, то можно нанести им еще больший ущерб, доведя его примерно до 11 %. Если же человек в течение недели употреблял по полторы чайные ложки имбирного порошка в день, то повреждения ДНК, вызванные окислительным стрессом, снижались примерно на 25 %, до 8 % клеток, что аналогично тому, что было обнаружено у людей, употреблявших такое же количество розмарина. Исследователи также проверили кумин, паприку, шалфей и куркуму. Первые три вида, по-видимому, не помогли, но куркума показала наилучший результат[2700].
Куркума
Еще одним распространенным компонентом традиционной окинавской кухни является куркума, которая увеличивает продолжительность жизни модельных организмов, включая дрожжи[2701], беспозвоночных и млекопитающих. Соединения куркумы привели к увеличению средней продолжительности жизни C. elegans на 39 %[2702], плодовых мушек – на 20 %[2703], мышей – на 12 %[2704], [2705], а также продемонстрировали антивозрастное действие на мозг пожилых крыс[2706].
В эксперименте по повреждению ДНК, в ходе которого люди в течение недели употребляли различные дозы специй, а затем подвергали свои клетки воздействию свободных радикалов, куркума оказалась лидером. У людей, получавших всего лишь щепотку куркумы в день, уровень повреждения ДНК снижался на 55 %. При этом использовался не патентованный экстракт куркумы, а примерно восьмая часть чайной ложки обычной специи, которую можно купить в любом продуктовом магазине. И это не было опытом в чашке Петри. Просто сравнивали, что происходит с клетками тех, кто еженедельно употреблял небольшое количество этой специи, и подсчитывали скорость разрушения ДНК[2707].
Подсчет количества повреждений ДНК в клетках до и после недельного употребления специй не выявил существенной защиты в группах имбиря и розмарина. Однако оказалось, что куркума снижает уровень повреждения ДНК примерно на 40 %[2708]. Возможно, это связано с тем, что куркума повышает активность собственных антиоксидантных ферментов организма. Каталаза – один из самых активных ферментов в нашем организме. Каждый из этих ферментов способен ежесекундно обезвреживать миллионы свободных радикалов. Если в течение месяца ежедневно употреблять примерно три четверти чайной ложки куркумы, то активность фермента каталазы в крови может повыситься более чем на 50 %[2709]. Ежедневный прием половины чайной ложки куркумы в соотношении 50: 1 с молотым черным перцем в течение 5 дней перед лучевой терапией снижает уровень окислительного повреждения примерно на 50 % по сравнению с контрольной группой[2710].
Обзор клинических эффектов и сомнений, связанных с куркумой, как обоснованных, так и не слишком, приведен в моем видеоролике see.nf/turmericskeptic. Рекомендую ежедневно принимать четверть чайной ложки куркумы.
Смузи с окинавскими мотивами
Я экспериментировал с рецептом восхитительного ярко-фиолетового смузи, который по вкусу напоминает тыквенный пирог. Сладкий картофель придает ему особенно шелковисто-гладкую текстуру. В моей готовящейся к изданию поваренной книге будет много рецептов, но для того чтобы разбудить ваш аппетит, предлагаю такой:
1/2 приготовленного, а затем замороженного фиолетового сладкого картофеля;
• кусочек корня куркумы примерно 0,5 см;
3/4 чайной ложки матча;
• 1 чашка несладкого соевого молока;
• 1 1/2 чайной ложки молотого льняного семени;
• 1 1/2 чайной ложки зародышей пшеницы;
1/4 чашки замороженной клюквы;
1/2 чашки замороженной клубники;
• 3 финика без косточек;
1/4 чайной ложки приправы для тыквенного пирога;
• щепотка кардамона.
Один фиолетовый сладкий картофель вымойте под проточной водой, затем несколько раз проколите вилкой. Приготовьте в микроволновой печи на высокой мощности, пока картофель не станет нежным. Когда картофель достаточно остынет, разрежьте его пополам и заморозьте обе половинки. (Половину вы используете для этого рецепта, а вторую половину – в следующий раз, когда вам захочется такого смузи). Поместите все ингредиенты в блендер и смешайте до однородности.
Советы. Чтобы сохранить корень куркумы, я нарезаю его на кусочки размером примерно полсантиметра и замораживаю. Кроме того, с тех пор как я узнал о спермидине (см. с. 38), я измельчаю льняное семя пополам с зародышами пшеницы, так что мне достаточно зачерпнуть одну полную столовую ложку смеси льна с зародышами пшеницы. Кроме того, обратите внимание, что количество матча (2 г), используемое в этом смузи, может содержать больше кофеина, чем порция эспрессо, поэтому не стоит пить смузи в конце дня.
Кто дольше?
По данным наиболее достоверных продолжительных исследований, в том числе «Оксфордского вегетарианского исследования»[2711], идеальный индекс массы тела (ИМТ), обеспечивающий наибольшую продолжительность жизни, составляет 20–22 (кг/м2)[2712]. Окинавцы традиционно находятся в середине этого диапазона и имеют стабильный индекс массы тела 21. Несмотря на культурную норму не наедаться до отвала, тот факт, что в среднем они потребляли около 1800 калорий в день, вероятно, в большей степени определяется качеством, а не количеством потребляемой пищи[2713]. На самом деле окинавцы съедали довольно много пищи, но цельные растительные продукты не слишком калорийны, и это фактически позволяло им придерживаться 11 %-ного ограничения калорийности[2714].
Такое мягкое и длительное ограничение потребляемых калорий, возможно, способствовало их исключительной выживаемости, хотя польза растительной диеты может превзойти пользу ограничения калорийности. Одна из популяций, которая живет еще дольше, чем японцы Окинавы, питается не на 98 %, а на 100 % без мяса[2715].
Адвентисты-вегетарианцы в Калифорнии имеют «возможно, самую высокую продолжительность жизни среди всех формально описанных популяций»[2716]. Мужчины и женщины доживают до 83 и 86 лет соответственно. Женщины-адвентисты живут примерно столько же, сколько и женщины Окинавы, а вот мужчины-адвентисты – дольше, чем окинавские мужчины[2717]. Лучшими из лучших оказались адвентисты-вегетарианцы, которые также занимаются спортом и не курят. Они живут в среднем до 87–89 лет. Это на 10–14 лет дольше, чем в целом по стране[2718].
Красно-бело-синяя зона
К сожалению, окинавское долголетие уходит в прошлое. На Окинаве сейчас расположено более десятка ресторанов KFC[2719], а уровень потребления окинавцами насыщенных жиров за годы, прошедшие после Второй мировой войны, вырос в 3 раза. Они прошли путь от практически полного отсутствия холестерина в ежедневном рационе до его количества, равного нескольким бигмакам[2720]. Они втрое увеличили потребление натрия и теперь испытывают такой же дефицит калия, как и американцы, получая менее половины рекомендуемой минимальной суточной нормы в 4700 мг. Всего за два поколения окинавцы превратились из самых стройных японцев в самых толстых[2721]. В связи с этим специалисты в области здравоохранения настойчиво рекомендуют окинавцам следовать окинавской диете.
То же самое можно сказать почти обо всех других «голубых зонах» – например, в Средиземноморье: они уже являются артефактами истории[2722]. Только одна «голубая зона» сохранилась и процветает до сих пор: я говорю об адвентистах седьмого дня в Лома-Линда, штат Калифорния. Ее специфика заключается в том, что она не имеет четких границ и окружена остальным обществом. Все остальные «голубые зоны» географически изолированы от материков, будучи островами, что позволяло сохранять уникальный рацион питания и образ жизни[2723].
Диета других «голубых зон» также была ограничена экономически: люди фактически были вынуждены питаться более здоровой пищей. Например, средний житель Окинавы не ел много мяса, сахара, соли, растительного масла и шлифованного белого риса, потому что просто не мог себе этого позволить[2724]. Окинава была самой бедной префектурой Японии[2725]. После Второй мировой войны в рационе жителей острова сладкий картофель стал составлять не более 5 %, а импортный белый рис и хлеб начали вытеснять более полезные продукты[2726]. В отличие от них, США – одна из самых богатых стран мира, со средним ВВП на душу населения, превышающим 65 тысяч долларов в год[2727], и при этом она занимает 45-е место по продолжительности жизни[2728]. Чему мы можем научиться у адвентистов из Лома-Линда, последней активной «голубой зоны» в США, община которой, похоже, превосходит все остальные по продолжительности жизни[2729]? Как им удалось сохранить свои привычки здорового питания в окружении излишеств современного мира?
Адвентисты придерживаются философии здоровья, основанной на библейском представлении о том, что к человеческому телу следует относиться как к храму[2730]. Поэтому уже более 140 лет они пропагандируют вегетарианство. Адвентисты уникальны тем, что большинство из них придерживаются диеты без мяса или с его минимальным употреблением[2731]. Так, например, в исследовании, в котором на протяжении более 10 лет принимали участие около 100 тысяч адвентистов Северной Америки, около 50 % являются вегетарианцами или веганами, а другая половина употребляет мясо в среднем лишь три раза в неделю[2732].
Религиозная жизнь
Адвентисты седьмого дня – это протестантская христианская деноминация. Может ли религиозность влиять на продолжительность жизни? По данным опроса тысячи взрослых американцев, 79 % респондентов считают, что вера может помочь людям излечиться от болезни[2733]. Может быть, в этом есть доля истины? Метаанализ более 70 исследований по этой теме показал, что глубина религиозности или духовности может быть связана со снижением смертности в здоровых популяциях, а также выявил признаки предвзятости в публикациях, что означает, что некоторые данные, не столь лестные для духовности, могли быть «заметены под ковер»[2734].
Даже если бы эта связь была устойчивой, существуют факторы, сбивающие настройки. Например, у неевангелических протестантов и иудеев самые низкие показатели смертности среди основных религиозных групп в США, но они также, скорее всего, белые, обеспеченные и хорошо образованные, а каждый из этих факторов сам по себе связан с большей продолжительностью жизни[2735]. Существует и обстоятельство, чреватое появлением обратной причинно-следственной связи. В этих исследованиях общим показателем веры является посещение религиозных служб, но очевидно, что больным посещать религиозные мероприятия затруднительно[2736].
Существуют исследования, говорящие о том, что религиозная активность связана с увеличением длины теломер[2737], хотя, как ни странно, самые длинные теломеры были обнаружены у наименее религиозных людей, например у тех, кто сообщал, что никогда не молился и не изучал священные книги, например Библию. Но среди тех, кто был хотя бы в некоторой степени религиозен, большая духовная вовлеченность была связана с большей длиной теломер[2738].
Религиозность, безусловно, может быть напрямую связана с показателями здоровья через выбор образа жизни: отказ от курения или чрезмерного употребления алкоголя, что, как правило, характерно для религиозных людей, или, в случае адвентистов, более здоровое питание[2739]. Может быть, склонность к более строгому соблюдению кодексов поведения помогает верующим придерживаться рекомендаций по здоровому образу жизни[2740]? Многие из советов адвентистов по питанию и образу жизни стали частью Complete Health Improvement Program (CHIP, недавно переименованной в Pivio) – наиболее подробно изложенной в медицинской литературе программы по коррекции образа жизни на уровне сообщества[2741]. (Подробно о ней читайте в книге «Не сдохни на диете».) Связь религиозной принадлежности и восприимчивости к программе была проверена на группе из 7000 участников.
Несмотря на то что адвентисты составляют менее 1 % населения США, примерно каждый пятый участник программы CHIP был верующим. Каковы же их результаты по сравнению с неверующими? Существенное снижение факторов сердечно-сосудистого риска было достигнуто как у адвентистов, так и у неадвентистов, причем у неадвентистов это снижение было более значительным. Исследователи пришли к выводу, что это свидетельствует о том, что адвентисты седьмого дня «не обладают монополией на хорошее здоровье»[2742].
Плодовитость против долголетия?
Адвентисты-вегетарианцы могут быть самыми долгоживущими, но какой ценой? Анализ спермы, проведенный в клинике репродукции Лома-Линда, вызвал вопросы к ее качеству. Хотя и оставаясь в пределах нормы[2743], количество сперматозоидов у вегетарианцев было примерно на 25 % ниже[2744]. У немногочисленных веганов концентрация сперматозоидов также была ниже, хотя и незначительно, но это компенсировалось на 30 % большим объемом эякулята. Однако у веганов было значительно меньше активированных сперматозоидов, что является признаком снижения фертильности. Исследователи предположили, что причиной может быть употребление сои, поскольку она может оказывать гормональное воздействие. Калифорнийские адвентисты-вегетарианцы в среднем употребляют около половины порции растительного мяса в день на основе сои. Фитоэстрогены сои были подвергнуты испытанию: несколько месяцев участники исследования употребляли сою в количестве, эквивалентном почти 20 порциям в день, и это не привело к негативному влиянию на параметры спермы[2745].
В исследовании участвовало всего пять веганов, поэтому результаты исследования качества спермы могут быть просто случайностью, но если они подтвердятся, то могут отражать эволюционный компромисс между фертильностью и продолжительностью жизни, впервые выявленный почти столетие назад[2746]. С помощью тонко настроенного лазера можно избирательно разрушать отдельные клетки в процессе развития C. elegan[2747] – и прекращение жизнедеятельности клеток, дающих начало сперматозоидам и яйцеклеткам, значительно увеличивает продолжительность жизни[2748]. Аналогичные результаты можно получить и на плодовых мушках, что позволяет сделать вывод: можно сместить приоритеты организма с размножения на выживание[2749].
Компромисс между плодовитостью и продолжительностью жизни может быть одной из причин, по которой стерилизация и кастрация наших питомцев может продлить их жизнь. По результатам исследования миллионов собак и кошек[2750], стерилизованные кобели и суки живут примерно на 20 % дольше, чем «интактные», стерилизованные кошки живут примерно на 40 % дольше, а кастрированные коты – на 60 % дольше[2751].
А как насчет кастрированных мужчин? Евнухи живут на 25 % дольше, чем некастрированные мужчины[2752]. В США люди, признанные «слабоумными», подвергались принудительной стерилизации десятками тысяч вплоть до 1950-х годов[2753]. Эту практику поддержал не кто иной, как знаменитый судья Верховного суда Оливер Уэнделл Холмс. Выступая в поддержку решения, принятого по делу «Buck v. Bell», – решения, узаконивающего евгеническую практику, – он писал: «Принцип, лежащий в основе обязательной вакцинации, можно расширить до перерезания фаллопиевых труб»[2754]. Отвратительная практика принудительной стерилизации все же позволила провести естественный эксперимент, и в одной из психиатрических клиник было обнаружено, что кастрированные мужчины живут в среднем на 14 лет дольше, чем интактные мужчины в той же больнице[2755].
Генеалогическая база данных, включающая около 200 000 мужчин и женщин и собранная за три столетия в 16 странах мира, показала, что те, кто имел меньше детей, живут дольше[2756]. Столетние люди, например, имели меньше детей и рожали их в более позднем возрасте[2757]. Это не означает, что меньшее количество детей позволит вам жить дольше, а скорее говорит о том, что конституциональные факторы, увеличивающие продолжительность жизни человека, могут быть достигнуты за счет снижения репродуктивного потенциала, что является еще одним примером теории антагонистической плейотропии (см. с. 118 и 157). Например, отбор модельных организмов для экспериментов по увеличению продолжительности жизни может привести к появлению более долгоживущих животных, но с пониженной плодовитостью[2758]. Это интуитивно понятно, если рассматривать ситуацию с учетом возможной нехватки пищи.
В неблагоприятные периоды для обеспечения долгосрочного выживания целесообразно отложить воспроизводство до наступления более благоприятных условий[2759]. Ограничение калорийности рациона может продлить жизнь животных, но при этом привести к снижению численности их потомства. Аналогичная картина может наблюдаться и у человека. В Миннесотском исследовании голодания испытуемые быстро теряли либидо после того, как калорийность их питания сокращалась вдвое[2760]. Помните пути старения, реагирующие на питательные вещества: AMPK, ИФР-1 и mTOR? Здесь могут быть качели между ускорением и воспроизводством тканей, с одной стороны, и их сохранением и омоложением – с другой[2761]. К счастью, мы можем улучшить баланс с помощью диеты.
Чем позже у девочек начинается менструация, тем дольше они живут. Каждый год задержки первой менструации значительно снижает риск смерти от сердечно-сосудистых заболеваний[2762], рака[2763] и инсульта, достигая наименьшего уровня общей смертности среди тех, у кого менструация началась только в 15 лет[2764]. Более раннее развитие молочных желез (до 10 лет против двенадцати или тринадцати) связано с повышением риска развития рака молочной железы на 23 % в более позднем возрасте[2765], а более раннее начало менструации у девочек значительно повышает риск развития рака мочевого пузыря, молочной железы, толстой кишки, печени, легких, кожи и матки[2766].
Если столетие назад первая менструация в среднем начиналась почти в 17 лет[2767], то сейчас в 12 лет и ранее[2768]. Аналогичным образом во всем мире за последние полвека возраст начала развития молочных желез снижался в среднем примерно на три месяца за десятилетие, доходя в США всего лишь до 9–10 лет, что потребовало изменения в учебниках определений «преждевременного полового созревания»[2769]. Но это то, что мы в определенной степени можем контролировать.
Более высокий уровень ИФР-1 связан с более ранним половым созреванием[2770], поэтому неудивительно, что у детей, потребляющих больше животного белка, половое созревание наступает значительно раньше; в случае с растительным белком такого эффекта не наблюдается[2771]. Метаанализ шестнадцати исследований, посвященных изучению питания и развития, показал, что каждый дополнительный грамм ежедневного потребления животного белка в детстве сдвигает первую менструацию на 2 месяца[2772]. Так, например, у семилетних девочек, потребляющих более 12 порций мяса в неделю, вероятность начала менструации в ближайшие 5 лет на 75 % выше по сравнению с семилетними девочками, потребляющими менее 4 порций мяса в неделю[2773]. Связь обнаружена для потребления как красного мяса, так и птицы[2774]. ИФР-1 и другие пути старения, однако, не могут полностью объяснить эти результаты, поскольку стойкие загрязнители, накапливающиеся в мясе, такие как ДДТ[2775], также были связаны с преждевременным половым созреванием[2776].
Растительная диета
Основным компонентом, который считается ответственным за необычайное долголетие адвентистов «голубой зоны» в Калифорнии, является их растительная диета[2777]. Адвентисты-вегетарианцы не только живут дольше, чем адвентисты-невегетарианцы, которые едят относительно мало мяса, но и имеют более низкую частоту всех раковых заболеваний, вместе взятых, а также меньше страдают от высокого кровяного давления и диабета[2778]. В целом комплексный метаанализ и систематический обзор основных обсервационных исследований, посвященных изучению растительного питания и хронических заболеваний, показал, что вегетарианская диета успешно защищает от сердечно-сосудистых заболеваний и смерти в результате них, а также от разных видов рака, причем веганская диета снижает риск развития рака вдвое[2779].
А как быть с теми, кто решил отказаться от вегетарианства и начать есть мясо? Исследование Adventist Health Study показало, что по сравнению с теми, кто оставался вегетарианцем, у людей, начавших есть мясо, риск набрать вес увеличивался примерно на 230 %, риск развития диабета – на 170 %, риск инсульта или сердечно-сосудистых заболеваний – на 150 %[2780]. А если они продолжали есть мясо, то продолжительность их жизни сокращалась на 3,6 года. Сопоставимое преимущество в плане выживаемости было обнаружено у вегетарианцев «со стажем». Продолжительность жизни тех, кто не употреблял мясо в течение 17 и более лет, составила 86,5 года, а тех, кто был вегетарианцем менее 17 лет, – 82,9 года[2781]. По сравнению с вегетарианцами у тех, кто употреблял в пищу любое мясо, птицу и рыбу, вероятность развития деменции оказалась в 3 раза выше[2782].
Люди хотят жить долго и при этом иметь гарантии, что их психическое и физическое здоровье будет отменным до глубокой старости[2783]. Помимо преимущества в долголетии, адвентисты-вегетарианцы не только живут дольше, но и отличаются более крепким здоровьем, что подтверждается меньшим количеством принимаемых лекарств, рентгеновских исследований, хирургических операций и госпитализаций. Кроме того, для них характерно более высокое качество жизни благодаря меньшему количеству хронических заболеваний[2784]. Исследование, проведенное среди 15 000 американских вегетарианцев, показало, что у них значительно реже встречается ишемическая болезнь сердца, меньше инсультов, случаев повышенного кровяного давления, диабета, дивертикулеза, аллергий и значительно меньше заболеваний в целом. Исследователи также отметили, что невегетарианцы чаще подвергались операциям по поводу различных заболеваний – от варикозного расширения вен и геморроя до гистерэктомии, а также принимали множество различных лекарств. Те, кто ел мясо, примерно в 2 раза чаще принимали аспирин, снотворные, транквилизаторы, антациды, болеутоляющие, препараты от давления, слабительные и инсулин.
Отказ от животной пищи приводит к значительному снижению расходов на медицину. По сравнению с невегетарианцами, которые также не курят и не пьют, у вегетарианцев значительно снизились траты на стационарное, амбулаторное и общее медицинское обслуживание, в том числе почти на 50 % – на лечение депрессии[2785]. Участники исследования, перешедшие на рацион на основе растений, отмечают улучшение не только самочувствия, но и настроения в сравнении с теми, кто продолжает придерживаться обычной диеты, что побуждает первых следовать такой модели питания в течение длительного времени[2786].
Уже через несколько дней или недель после перехода на растительную диету пациенты отмечают очевидные преимущества растительного питания, в том числе улучшение таких показателей, как уровень сахара в крови и вес, и это создает дополнительную мотивацию для продолжения[2787]. Более того, иногда растительная диета оказывалась настолько приятной и эффективной, что люди, принимавшие участие в исследовании и переведенные на растительную диету на время, по окончании эксперимента отказывались возвращаться к прежним моделям питания[2788].
Как вы считаете, что действеннее: просить пациентов внести серьезные изменения в рацион или незначительные? Парадоксально, но исследования диеты показали, что глобальные перемены приводят и к высоким результатам. Исследователи сделали вывод: «Возможно, стоит заменить распространенный совет “умеренность во всем” на “большие усилия – большая победа”»[2789].
Замена мяса
В США фактором риска смерти номер один является американская диета. Ежегодно около полумиллиона матерей, отцов, сестер, братьев и друзей умирают от сердечно-сосудистых заболеваний только из-за того, что они едят[2790]. Только сравните: растительная диета связана с более низким риском развития сердечно-сосудистых заболеваний, более низким риском смерти от сердечно-сосудистых заболеваний и, фактически, более низким риском смерти от всех причин, вместе взятых. Постепенное увеличение потребления растительной пищи за счет снижения количества животной пищи может позволить нам прожить более долгую и здоровую жизнь[2791] – при этом ничем не жертвуя[2792].