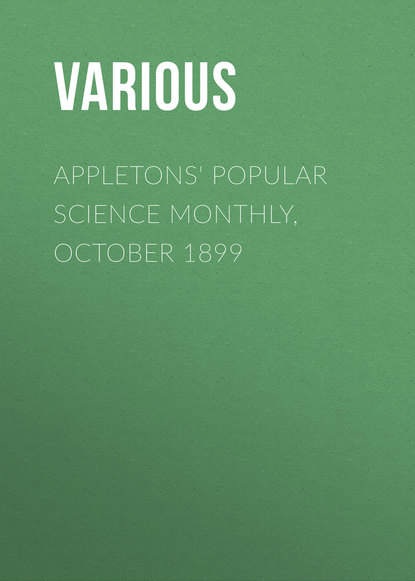
Полная версия:
Appletons' Popular Science Monthly, October 1899
Do we do anything of this kind? When I was taught to read and write I knew how to write the figure 2 before I had any idea of the number two. Nothing is more radically contrary to the normal working of the brain than this. The notion of numbers – up to 10, for example – should be given to the child before accustoming him to trace a single character. That is the only way of impressing the idea of number independently of the symbol or the formula which is only too ready to take the place in the mind of the object represented by it.
When a child has learned to count through the use of such objects as I have mentioned he may be taught what is called the addition table. This table can be learned by heart easily enough, but when we reach the multiplication table we come upon one of the tortures of childhood. Would it not be simpler and easier to make the children construct these tables, instead of making them learn them?
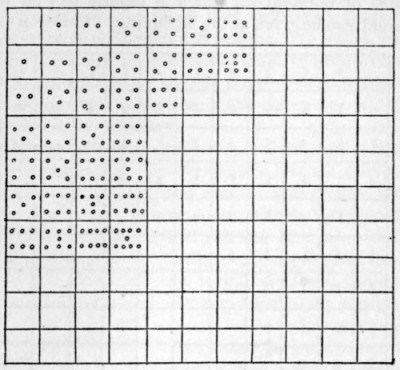
Fig. 1.
Let us first take the addition table, and suppose that we trace ten columns on suitably ruled paper, at the top of which we write the first ten numbers, for example, and then write them again at the beginning of a certain number of horizontal lines (Fig. 1). Let us suppose, too, that we have a box divided into compartments arranged like the squares in our table, into which we put heaps of balls, beans, or dice corresponding to the numbers indicated in the table. The child will take, for example, two balls from one compartment and three from another, will put them together and place his five balls in the case corresponding with the point where the lines of two and three will meet, and will thus gradually accustom himself to the idea that two added to three are equal to five, four and two to six, etc., before he knows how to write the corresponding figures. As soon as he has learned how to write them he can himself make the table with figures (Fig. 2), showing that one and one make two, one and three four, etc.
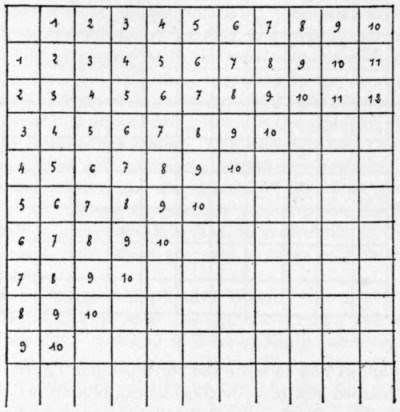
Fig. 2.
This will be all the easier for him because he will only have to write the figures in their order in the lines and the columns. This furnishes an excellent writing exercise after the children have begun to write figures, and affords besides a certain method of teaching them the addition table up to nineteen at least. I insist that all this can be done even before the child knows how to write the figures by means of an arrangement like a printer's case, and that it will be as a play, rather than a study, to the child. Hardly anything more will be required than to bring the toy to the child's notice and leave him to himself after he has been started with it, and he will get along the faster the less he is bothered.
A similar process may be adopted with the multiplication table. With a case like the other, it is only necessary to tell the child that if he wants to know how much are three times four he has only to make heaps of four things each, take three of them and put them in the box at the intersection of the line three and the column four. If he can write the figures he will write 12, instead of gathering up the twelve objects that represent the product. When he has played at this for some time he may become acquainted with all the products up to ten times ten or beyond without having to make any abnormal effort of memory.
The idea of numeration, which is usually put off till a later period, should also be given at the beginning. Children soon understand the decimal numeration and learn to write 10 for ten, and other numbers composed of one of the nine ciphers and zero. But the fact which, however, though quite essential to know, receives very little attention is that there is nothing particular about this number ten, and that systems of numeration can be devised resting on any basis that may be taken; that the principle of every system of numeration consists in taking a certain number of units and grouping them. Take, for example, a system having five as its basis. All the numbers of such a system can be represented with the figures 1, 2, 3, and 4, the symbol 10 standing in this case for five. To construct a number we have only to group the units by fives and observe the result.
To learn decimal numeration by this process we put tens of objects into little boxes, tens of little boxes into larger ones, and so on. The child can in this way acquire an exact idea of the units of successive order in any system that may be desired.
This method of teaching was developed in a remarkable way about thirty years ago by Jean Macé in a little book entitled L'Arithmétique du Grand-Papa– Grandpa's Arithmetic – which made some impression when it appeared, but has been substantially forgotten.
In this method I attach much importance to giving these exercises a form of play. I believe that nothing in primary instruction should savor of obligation and fatigue. It would, on the other hand, be better to try to induce the child to desire himself to go on, and it would always be well to try to give him the illusion, in all stages of instruction, that he is the discoverer of the facts we wish to impress upon his mind.
We need not stop with arithmetic, but may go on and give the child a little geometry. To accomplish this we should give him the idea of geometrical objects, and to some extent their nomenclature, and this can be done without causing fatigue. To accomplish this he should be taught to draw, however rudely. He can begin with straight lines, of which he soon learns the properties; then, when he has drawn several lines side by side, he will learn that they are parallels and will never meet. He will learn, too, after he has drawn three intersecting lines, that the figure within them is called a triangle, that the figure formed by two parallel lines meeting two other parallels is a parallelogram, and he can go on to make and learn about polygons, etc (Fig. 3). All this nomenclature will get into his head without giving abstract definitions, but in such a way that when he sees a geometrical object of definite form he will recognize it at once and give it the name that belongs to it.
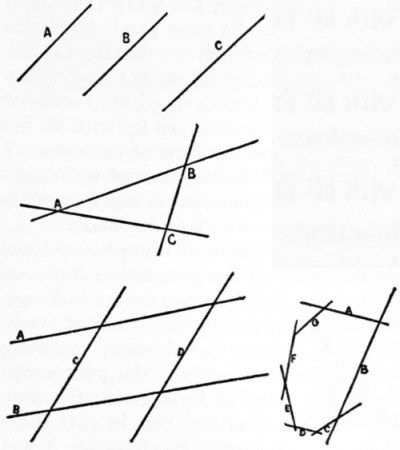
Fig. 3.
In the practical matter of the measurement of areas we convey immediate comprehension as to many figures without special effort, provided we do not present the demonstration in professional style, limiting ourselves to making the pupil comprehend or feel things so clearly and definitely that it shall be equivalent, as to the satisfaction of his mind, to an absolutely rigorous demonstration. At any rate, he will be better provided for the future than by rigorous demonstrations that he does not understand. Taking the parallelogram, for example, let us suppose a figure made like Fig. 4, and we saw through it along the lines A A' and B C. It does not need a very great effort of attention to recognize, experimentally if need be, that the two triangles A A' D and B B' C may be placed one upon the other and are identical. If, from the figure thus formed, we take away the right-hand triangle the parallelogram will remain; if we take away the other triangle a rectangle will be left, or a peculiar parallelogram, of which also we give the idea to the child as a figure in which the angles are formed by straight lines perpendicular to one another. Here, then, the child gains the notion of the equivalence of a parallelogram and a rectangle of the same base and height; and this notion, obtained by cutting up a piece of board or pasteboard, he will carry so seriously and firmly in his head that he will never lose it. By cutting the same parallelogram in two, along a diagonal A C, it may be easily shown that the two triangles can be placed exactly one upon the other, and that, consequently, they have equal areas. These lessons constitute a series of classical theorems in geometry which the child can try with his fingers and learn without even giving them the form of theorems. I might show the same as to the area of the trapeze and with many other theorems, but my purpose is only to present as many examples as will make my idea understood, without going into details.
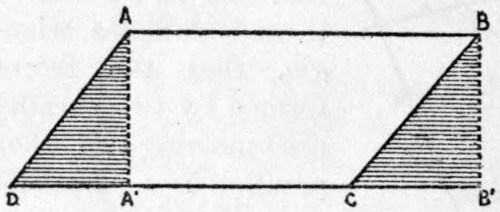
Fig. 4
Yet I can not leave this subject without showing how we can make a very child understand some of the geometrical theorems that have acquired a bad reputation in the world of candidates for degrees, including even such as the pons asinorum of Pythagoras; the demonstration, that is, that if we construct the triangles B and C on the sides of a right-angled triangle, their sum will be equal to the square A constructed on the hypotenuse. The usual demonstration of this theorem is not very complicated, but there is something tiresome, artificial, and hard in it. The demonstration I propose is almost intuitive, and the reasoning of it is both simple and rigorous.
Suppose we take two equal squares, and, making equal lengths on the four sides of one of them, join the points so obtained as indicated in the first of the two figures (Figs. 5 and 6) so as to form four right-angled triangles, and then place four other squares in the corners of the original square. These right-angled triangles are of such sort that the sum of their sides is equal to the side of the square. This can be demonstrated, but it strikes the eyes without that. We see, too, that the interior figure is a square, and that it is constructed on the hypotenuse of the triangles in question.
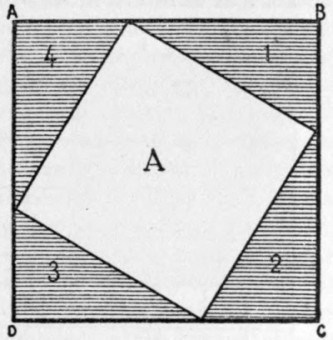
Fig. 5.
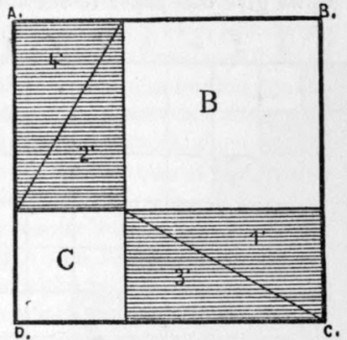
Fig. 6.
It is easy to see in the other figure, which is formed after the same measures as its alternate, that the triangles 1, 2, 3, 4 can be arranged so as to occupy the positions 1', 2', 3', 4' in such way as to leave in the main square two smaller squares constructed on the sides of one of the right-angled triangles. It follows that the square A is equivalent to the sum of the squares B and C. The theorem thus becomes a kind of intuition, a thing evidently indisputable.
It is a curious fact that the origin of this demonstration is lost in the obscurity of the past; it probably goes back to thirty or forty centuries, at least, before the Christian era, and apparently to India. Bhascara, in his Bija Ganita, after tracing a figure, a simple combination of these two, says, "There you see it." I remark that such a demonstration, even if dressed with geometrical terms, assuming a character that conforms to existing ways of teaching, would be vastly superior, even in secondary schools, to the demonstrations of Legendre and others, which are much harder. The return to what was done very long ago in this case constitutes a great advance upon what we are doing now.
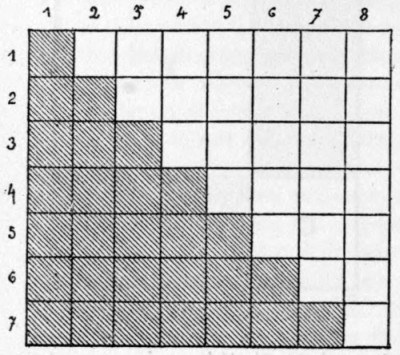
Fig. 7.
Having given our little one an initiation into the mysteries of arithmetic and geometry, we introduce him to algebra, a branch which passes in the majority of families as the hardest, most complicated, and most abstruse that can be imagined. I do not pretend that algebraic theories enter easily into the child's delicate brain; rather the contrary; but I declare that some ideas in algebra can be made comprehensible to children without fatigue. We can, for instance, make them understand, in the way of amusement and without great difficulty, the formula that gives the sum of the first numbers. We take a sheet of paper ruled in squares and shade the first square of the first line, then the first two squares of the second line, the first three of the third, etc. (Fig. 7). The whole number of squares shaded in this manner represents visibly the sum of the first whole numbers up to any one we may choose – to 7 in the figure. If we give this paper to the child and ask him to return it, he will very easily perceive that the figures formed by the white and the black squares are alike. The number sought for will therefore be equal to half the sum of the squares – that is, in the present example
1 + 2 + 3 + 4 + 5 + 6 + 7 = (7 X 8): 2 = 28,we can prove by reasoning that if n be taken to represent the last number we shall have for the sum
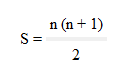
I introduce this formula to define my thought better, but one can make the child perceive the numbers that are wanted without writing down a single character.
Somewhat similar is the method of finding the sum of the odd numbers. For this it will be enough to take our square-ruled sheet of paper and shade the first square on the left, then the three squares around it, which will form with it a square (1 + 3 = 4); continuing thus we obtain, as the figure readily shows (Fig. 8), a square formed of a series of shaded zones, representing the series of odd numbers, the examination of which will illustrate the property to the child.
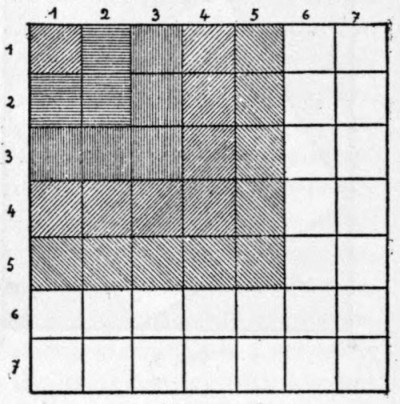
Fig. 8.
In another direction it is possible to give the child algebraic ideas much beyond anything we would imagine. Suppose, for example, we want to give him a conception of addition. He easily realizes that objects – material bars, for example – can be selected so as to represent numbers by their length. He can be readily made to understand that if he has one bar three and another five inches long he can obtain the sum of these lengths, in what we might call a material way, by placing them lengthwise, one at the end of the other – an essentially practical notion and easily carried into effect. If we take a line and mark a starting point on it, calling it zero, then measure off segments on it representing the bars we have been talking about one after another, we can get the sum represented by the length of the two segments. If, instead of measuring three plus five inches I measure three plus two I reach another point. If, instead of adding two and three, I wish to take one of the bars or numbers away (3-2), or subtract, the operation will be easily performed by measuring the two in the opposite direction. The difference will be represented by the length that is left. If we try to form the quantity 3-5 in arithmetic we can not do it; but in proceeding in this method and measuring back on the bar we get to a point back of the original starting point which represents this difference – say two inches behind where we began. Here we have in the germ the whole theory of negative quantities, concerning which thousands and thousands of pages have been written. Yet we find that by carefully graduating our lines we can make it intuitive and accessible to a child who has learned that the common operations of addition and subtraction can be represented with material objects. The generation of negative and positive quantities follows quite naturally.
These examples, I think, are sufficient to show that we might considerably enlarge the field of the investigations within reach of the child. For this purpose a small amount of very simple material, which we can vary as we please, is needful. The first element of this material is paper ruled in squares, a wonderful instrument, which everybody dealing with mathematics or with science generally should have. It is of special pedagogic use in giving children their first ideas of form, size, and position, without which their early instruction is only a delusion. Add to this paper dice, buttons, beans, and match-sticks – things always easy to get – and we have all the material we need.
There is no amusement, however puerile it may appear, not even a play of words, that can not be utilized in teaching of this sort. For instance, when your child has learned his addition table, if you put him to a demonstration, assuming to prove to his comrades that six and three make eight, his curiosity will be excited, and you may be very sure that, once his attention has been given to this amusement, he will never forget that six and three make nine and not eight. To make the demonstration, we have only to group the nine match-sticks as in the figure (Fig. 9) below. We might demonstrate in a like way that half of twelve is seven by cutting the Roman numeral XII in two, leaving the upper part visible. Such pleasantries have a pedagogical value, because the paradox is precisely of a kind to attract the attention of the child, and he will always afterward be sure not to fall into the trap.
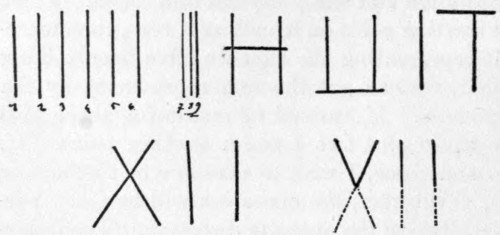
Fig. 9.
The side of this kind of instruction on which I insist most is that, given under the form of play, it is free from every sort of dogmatic character. No truth should be imposed on the child; on the contrary, he should be allowed to discover it as a fruit of his own activity. He will be thoroughly impressed with the truths which he has thus found out himself. They had better be few at first; the important thing is for him to know them completely.
The instruction should also be essentially objective and free from all abstraction. The absence of abstraction should, however, be rather apparent than real. Abstraction is indeed one of the elements that contribute most to give mathematical science a fearful air to outsiders, and yet it is most usually a simplification of matters – quite the contrary of what is generally supposed. It is, in fact, such a simplification and so necessary that we all make it as if by instinct, and the child makes it, not in mathematics only, but in all the considerations of life.
Thus, when I want to give the child his first idea of the number two I put two beans in his hand and let him contemplate them. He gets a perfect notion of the collection two. Yet, if you look at them a little closer and he himself looks at them closer he will find that the two beans, whatever else they may be, are not identical, for there exist no two objects in Nature that are not different. So when the child introduces this idea of collection into his mind in a wholly instinctive way, by identifying the things he sees, he begins to perform abstraction. This abstraction delivers him from all the complications and all the annoyances that come to him from the contemplation of real objects. By the philosophic process of abstraction it has been possible to construct all the sciences, and especially the science of magnitudes.
The ideas I have been setting forth in outline are not mine, and are, unfortunately, not recent. They may be found in somewhat different form, but substantially the same in principle, in l'Essai d'education nationale, published by Le Chalotais in 1763. The paper furnishes a programme of studies and education which, if put into execution, would, I believe, constitute a long advance over the present conditions. At a later period Condorcet was occupied with the subject. At the close of the nineteenth century the name of Jean Macé, which I have already cited, should be held among those of men who have tried to infuse sound and just views concerning the pedagogy of mathematics. Another man, from whom I have borrowed a considerable part of the examples I have cited, is Edouard Lucas, who, in his Récréations mathématiques, of which one volume was published during his lifetime and two others after his death, and in his lectures before the Conservatoire des Arts et Métiers, strove to develop views concerning the primary mathematical education of childhood – views which did not differ, except in form, from those which I have presented. —Translated for the Popular Science Monthly from the Revue Scientifique.
PRESENT POSITION OF SOCIOLOGY
By F. SPENCER BALDWINThe present condition of sociological thought is confused, if not chaotic. It needs only a brief examination of the writings of professed sociologists to discover the want of agreement among them. There is no consensus of opinion regarding either the scope and method of the new science, so called, or its fundamental laws and principles. The name sociology stands for no definite body of systematic knowledge. It is applied to an inchoate mass of speculation, often vague and conflicting, which represents the thought of various thinkers about social phenomena.
A few years ago a student of sociology in Chicago wrote to "all the teachers of sociology in the United States, and to others known to be deeply interested in the subject and entitled to express an opinion," asking them to answer a number of pertinent questions regarding the nature and function of the "science."28 About forty replied; of these, three discreetly pleaded knowledge insufficient to entitle them to an opinion. Comparison of the views expressed in the remaining twenty-seven replies led the investigator to conclude that the science is in a more or less undefined and tentative position. So little progress toward unanimity of opinion has been made by sociologists since the date of this census that its results may be taken as typical of present conditions. Among the questions asked were these: "Do you think the study is entitled to be called a science?" "In what department does it belong?" "What is its relation to political economy, history, political science, ethics?"
The question whether sociology is entitled to be called a science is answered by "fully three fourths" of the correspondents in the affirmative. Some hedge, by affirming that it is "becoming a science." Prof. John Bascom, of Williams College, appears to have entered into the humor of the situation; he writes, "It will do no harm to call it a science if we do not abate our effort to make it one."
The opinions regarding the department in which sociology belongs are entertainingly diverse. Prof. John Dewey, of the University of Chicago, is frank enough to admit that he doesn't "feel at all sure" where it belongs. "It would seem well," he adds, "to have it a separate branch, in order to make sure that it received proper attention." This feeling of uneasiness lest the claims of sociology be slightingly treated appears to be general among the representatives of the new study. Most of the teachers of sociology are of the opinion that it ought to form a department by itself. Some would place it in the department of the social sciences, along with politics, economics, jurisprudence, and the like. Others would change the order, making all the social sciences divisions of sociology. On the other hand, Professor Giddings, of Columbia University, says: "General sociology can not be divided into special social sciences, such as economics, law, and politics, without losing its distinctive character. It should be looked on as the foundation or groundwork of these sciences, rather than as their sum or as their collective name." Scattering replies place it under psychology, moral and political science, political economy, and anthropology. One teacher thinks it belongs under the "humanities"; while two say it has no natural boundaries, and is therefore not included in any one department. Altogether the impression left by the replies to this question is that the teachers of sociology are quite at a loss to know where to put the study in the university curriculum. They appear to realize confusedly that they have on their hands a pedagogical white elephant, which defies classification.
The opinions concerning the relation of sociology to political economy, history, political science, and ethics are almost delphic in their vagueness. Says one, "History is its material, ethics its guide, political economy its interpreter, and a rational system of political science its proposed end." Says another, "Sociology is political economy in practice, history in the making, political science as an art, and ethics applied." After worrying over these oracular epigrams it is refreshing to be told by another teacher that "the relation of sociology to political economy, history, etc., is close."