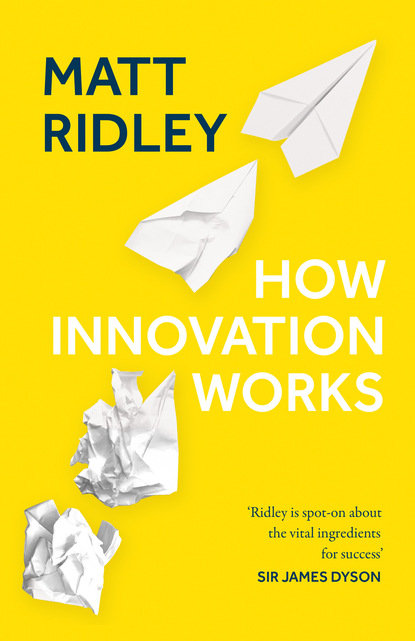
Полная версия:
How Innovation Works
Acting perhaps on a hunch, Pasteur now turned to a virulent cholera strain that normally killed chickens easily and injected it into these now recovered (and long-suffering) birds. It failed even to sicken them, let alone kill them. The weak strain of cholera had immunized them against this stronger strain. Pasteur began to realize that vaccination worked by a less virulent organism triggering an immune response that worked against a more virulent one. Not that he understood yet the slightest thing about the human immune system. Science was beginning to catch up with technology.
The chlorine gamble that paid off
The scene is a court house in New Jersey and the year is 1908. On trial is the Jersey City Water Supply Company, which had lost a previous case in which it had been proved that the company was not supplying ‘pure and wholesome water’ to the city, as specified by its contract. The problem was that upstream of the city’s reservoir more and more people were building homes and discharging sewage from privies directly into the streams that fed the reservoir. Deaths from typhoid were far too common in the city. Despite removing more than 500 such privies since 1899 and filtering the water, the company could not prevent the contamination of the water supply happening two or three times a year after heavy rain.
Given three months to rectify the situation by the court, the company’s sanitary adviser, Dr John Leal, came up with the idea of dripping chloride of lime, a disinfectant, into the water supply. By 26 September, three days before the second trial began, the plant was built, operating and continually chlorinating 40 million gallons of water a day. It emerged during the trial that Leal had sought nobody’s permission to conduct this experiment on the citizens of Jersey City, at a time when there was widespread revulsion at the idea of putting chemicals into drinking water. ‘The idea itself of chemical disinfection is repellent,’ thundered the suitably named Thomas Drown of the Massachusetts Institute of Technology, echoing the views of others in the establishment. Dr Leal’s decision was brave and risky.
In court, the city’s lawyer therefore objected to the notion that the company could meet its responsibility to provide clean water with a quick chemical fix of unknown risk to the population – which had not been asked for its consent. He requested the judge refuse even to hear the evidence about whether chlorination had helped. The judge disagreed and allowed the company to present its case. Under cross-examination, Leal said of chlorination: ‘I think that it is the safest, the easiest, and the cheapest and best method for rendering this water pure every day in the year and every minute of every hour.’ He added: ‘I believe the water supply of Jersey City today is the safest in the world.’
Q. Any ill effect on the health of the people there?
A. Not the slightest.
Q. Do you drink this water?
A. Yes, sir.
Q. Habitually?
A. Yes, sir.
After a lengthy trial the judge eventually ruled that the company had met its responsibilities by this innovation. The Jersey City case proved a turning point, a clean-water-shed. Cities all over the country and the world began using chlorination to clean up water supplies, as they do to this day. Typhoid, cholera and diarrhoea epidemics rapidly disappeared. But where did Dr Leal get the idea? From a similar experiment in Lincoln in England, he said at the trial. Like most innovators he did not claim to be the inventor.
The city of Lincoln had seen death rates from typhoid decline after the installation of a sand-filtration plant for its water supply. But in 1905 it was struck by a bad outbreak: 125 people died. The city called in Dr Alexander Cruickshank Houston, a bacteriologist with the Royal Commission on Sewage Disposal. Within two days of arriving in February 1905, Dr Houston had jerry-rigged a device for dripping Chloros (sodium hypochlorite) by gravity into the water, with immediate results on the rate of new typhoid infections.
But where did Dr Houston get the idea? Perhaps from an Indian Army Medical Services officer by the name of Vincent Nesfield, who published a paper in 1903 suggesting exactly how to make and use liquid chlorine to disinfect water supplies. Nesfield’s technique is close to what is used today, and well ahead of its time. Whether and where he ever used it is unknown.
And where did Dr Nesfield get the idea? Perhaps from a typhoid outbreak in Maidstone in Kent in the autumn of 1897, in which 1,900 people caught the disease and about 150 died. Here, ‘under the supervision of Dr Sims Woodhead, acting on behalf of the water company, the reservoir and mains of the Farleigh area of water supply at Maidstone were on Saturday night disinfected with a solution of chloride of lime.’ By December the outbreak was over.
And where did Dr Woodhead get the idea? Probably from the use of chloride of lime as a disinfectant of sewage, which was by then a well-known technique. Chloride of lime had also by this time caught on as an antiseptic among surgeons, though they were disgracefully slow as a profession to realize that they should be washing their hands at all let alone with strong bleach.
During London’s cholera epidemic of 1854, chloride of lime was used so liberally in Soho that, as one magazine reported, ‘The puddles are white and milky with it, the stones are smeared with it; great splashes of it lie about in the gutters, and the air is redolent with its strong and not very agreeable odour.’
At the time of that London epidemic, Dr John Snow was trying, largely in vain, to persuade the authorities that cholera was caused by dirty water, not smelly air – the ‘miasma’ theory then in vogue. He had shown that those getting their water supplies from the Thames estuary were far more likely to catch cholera than those getting their supply from rural streams, and he famously removed the handle from a water pump in Broad Street in Soho around which a cluster of cholera cases had developed.
But he was widely ignored, and chlorine was being spread in the streets for the wrong reason: to combat the supposedly dangerous smell, not to kill water-borne germs. In the ‘great stink’ of 1858, when parliamentarians were so disgusted by the smell from the River Thames that they at last authorized the construction of modern sewers to carry the sewage out to sea, chloride of lime was applied to window blinds in Parliament to mask the smell.
So the source of the invention of chlorination, like that of vaccination, is enigmatic and confused. Only in retrospect can it be seen as a disruptive and successful innovation that saved millions of lives. It evolved rather slowly, probably from serendipitous beginnings in largely mistaken ideas.
How Pearl and Grace never put a foot wrong
In the 1920s the most lethal disease to affect American children was whooping cough, or pertussis. It killed about 6,000 children a year, more than each of diphtheria, measles and scarlet fever. There were vaccines for whooping cough available in some places but they were almost useless. The only preventive was quarantine and even this worked poorly since nobody knew how long it was necessary to isolate the victims for. It was this problem that drew a pair of ordinary but extraordinary women, both of whom had begun their careers as teachers, into research on the disease.
Pearl Kendrick, from New York State, had studied bacteriology in 1917 at Columbia University, while working as a teacher. By 1932 she was at the Michigan state public health laboratory in Grand Rapids, busily analysing the safety of water and milk. That year she recruited Grace Eldering, who was originally from Montana and had likewise turned from teaching to bacteriology, to join her team. At the time an outbreak of virulent whooping cough was sweeping through the city, and Kendrick asked her boss if she could work on it in her spare time. She and Eldering set out to develop a reliable test for who was infectious. This was a ‘cough plate’ of the medium in which the pertussis bacterium would grow, and on to which patients would cough. If the bacteria grew, then the patient was infectious.
Laboriously manufacturing their own medium for the cough plates and going out to homes all over Grand Rapids, to collect samples, at the end of long days of paid work, Kendrick and Eldering had their eyes opened to the deprivation that had worsened the plight of the working class since the start of the Depression. By the light of kerosene lamps, they saw children struggling for breath in workless and hungry households. Quarantine sometimes meant destitution for a family where the breadwinner could not then go out to work. Soon they had established that most people were infectious for four weeks, which helped influence local and national policy on quarantine. But they wanted to go further and develop an effective vaccine.
And this they did, systematically and gradually, by using standard vaccine development techniques, over the next four years: nothing new or really clever, just careful experiments. The end result was a killed version of several strains of the bacterium that, when injected into mice, guinea pigs, rabbits, and Kendrick’s and Eldering’s own arms, proved safe. Now to show that it protected people against whooping cough.
Here the two scientists proved to be adept at the social as well as the laboratory side of the work. They did not want to do what was typical at the time and use as a control group orphans who would be denied the vaccine to show that it worked, but they needed to match those who received the vaccine with similar people who did not receive it. With the help of local doctors and social workers they used the Kent County Welfare Relief Commission’s statistics to identify a selection of people who matched those given the vaccine in age, sex and location but who had missed out on the vaccine for whatever reason. During 1934–5, they found that four of the 712 vaccinated children caught whooping cough, whereas forty-five of the 880 unvaccinated controls went down with the disease.
When Kendrick and Eldering announced these results at the annual meeting of the American Public Health Association in October 1935, the audience was sceptical, suspecting that the trial was faulty in some way – as many trials were in those days. A doubtful medical scientist named Wade Hampton Frost came twice from Johns Hopkins to examine the methods employed but eventually admitted that he could find nothing wrong with the two women’s work. At the same time, Kendrick wrote to Eleanor Roosevelt, inviting her to visit the laboratory, and to her amazement received an acceptance. The First Lady spent thirteen hours with the two scientists and went back to Washington to persuade the administration to find ways to fund the project so they could hire more people to help. This enabled Kendrick and Eldering to do a second, larger trial, using just three injections per child instead of four, to which large numbers of families immediately applied. In 1938, when the second trial produced even stronger results, Michigan began mass-producing the vaccine and by 1940 the rest of the country had followed suit, followed by the rest of the world. Whooping cough incidence and mortality fell rapidly and permanently to very low levels.
Kendrick and Eldering received very little recognition for this work, turning down most requests from the media even decades later, and little financial reward. They shared their methods and formulae freely all over the world. They did everything right: chose a vital problem, did the crucial experiments to solve it, worked with communities to test it, gave it to the world and wasted no time or effort defending their intellectual property. When not travelling to spread the message and the vaccine, they lived together in a house in Grand Rapids and threw generous parties and picnics for their co-workers. Nobody had a bad word to say of them. As one colleague said later: ‘Dr. Kendrick never became rich and, outside a relatively small circle of informed friends and colleagues, never became famous. All she did was save hundreds of thousands of lives at modest cost. Secure knowledge of that fact is the very best reward.’
Fleming’s luck
Fifty years after Pasteur’s summer holiday led to a fortuitous insight into the mode of action of vaccination, summer holidays would produce another piece of serendipity in the conquest of disease. Alexander Fleming left his laboratory in London to spend August 1928 in Suffolk. The weather that summer in London was changeable: cool in much of June, then suddenly rather hot in July, the temperature reaching a stifling 30ºC on the 15th, before cooling dramatically in early August, then heating up again after 10 August. This is of relevance because it affected the growth of the Staphylococcus aureus bacteria that Fleming was growing in petri dishes as he prepared a chapter for a book on bacteria. Though he was an expert on the species, he wanted to check some of his facts. The cold spell of early August was just right for the growth of a mould, of the fungus Penicillium, a spore of which had somehow floated into the laboratory on the wind and landed on one of the petri dishes. The hot spell that followed allowed the bacterial culture to expand, leaving only a gap around the Penicillium, where the mould had killed the staphylococci. It created a striking pattern, as if the two species were allergic to each other. Had the weather been different this pattern might not have been possible, because penicillin is not effective against mature bacteria of this species.
Fleming, a diminutive and taciturn Scot, returned from holiday on 3 September and – as was his habit – began inspecting the cultures he had left behind in the petri dishes cluttered into an enamel tray, before discarding them. A former colleague, Merlin Pryce, put his head around the door and Fleming engaged him in conversation as he worked. ‘That’s funny,’ he said when he picked up the plate with the pattern of exclusion between the fungus and the bacterium. Was the fungus producing a substance that killed bacteria? Fleming was immediately intrigued and saved both the plate and a sample of the fungus.
Yet it was to be more than twelve years before anybody turned this discovery into a practical cure for diseases. Part of the problem was the success of vaccination. Fleming’s career had been largely under the influence of a great pioneer of bacteriology, Sir Almroth Wright, who was convinced that diseases would never be cured by medications, however effective, but by assisting the body to defend itself. Vaccination should be used to treat, as well as prevent disease.
Wright, the son of an Irish father and a Swedish mother, was a towering figure, outspoken, eloquent and irascible. Among colleagues he was known as ‘the Praed Street Philosopher’, ‘the Paddington Plato’ or more mischievously Sir Almost Right or Sir Always Wrong. ‘Stimulate the phagocytes!’ was Wright’s battle cry, immortalized in Bernard Shaw’s play The Doctor’s Dilemma, in which Sir Colenso Ridgeon is a thinly disguised depiction of Wright. St Mary’s Hospital, where Wright and Fleming worked, became the high temple of vaccine therapy. Wright’s championing of typhoid vaccination for the Allied troops in the First World War probably saved hundreds of thousands of lives.
Influenced by Wright, Fleming’s scepticism that a chemical could ever be found to cure infections was reinforced by his experiences researching the causes of infection in wounds during the First World War. He and Wright were stationed in a casino in Boulogne, which they turned into a bacteriological laboratory, the better to understand how to save lives. Here Fleming showed, using test tubes deformed to resemble jagged wounds, that antiseptics like carbolic acid were counterproductive, because they killed the body’s own white blood cells without reaching the gangrene-causing bacteria deep in the crevices of the wounds. Instead, Fleming and Wright argued, wounds should be cleaned with saline solution. It was an important discovery, and one that doctors treating the wounded almost completely ignored, because it felt all wrong not to dress wounds with antiseptics.
Yet Fleming was not dogmatic about his devotion to Wright’s ideas. Before the war he had adopted the medical scientist Paul Ehrlich’s arsenic-based chemotherapy, Salvarsan, for syphilis and became renowned as a ‘pox doctor’; so he knew that there were other ways to treat disease than by stimulating the phagocytes. In 1921 he discovered the bacteria-killing properties of a protein, lysozyme, found in his own nasal mucus and in tears, saliva and other fluids – and that was secreted by phagocytes. The body’s own natural antiseptic, lysozyme hinted at the possibility of finding chemicals that could kill bacteria when injected into the body. But lysozyme itself proved disappointing against the most virulent species of bacteria responsible for disease.
Thus Fleming was at least partly prepared for the discovery of penicillin, or ‘mould juice’ as he initially called it. In a series of experiments he showed that it killed many kinds of virulent bacteria more effectively than most antiseptics, but did not kill the body’s own defensive phagocytes. But early experiments with penicillin as a topical antiseptic applied to infected wounds were disappointing. Nobody yet realized that it worked best if injected into the body. Also, it was hard to produce in quantities or to store. Notoriously, in 1936, the pharmaceutical company Squibb concluded that ‘in view of the slow development, lack of stability and slowness of bacterial action shown by penicillin, its production and marketing as a bactericide does not appear practicable.’ So penicillin languished as a curiosity, undeveloped as a cure for disease, for more than a decade. Fleming was a denizen of the laboratory, not the clinic or the boardroom.
It is generally assumed that it was the outbreak of war that accelerated the development of antibiotics, but the evidence suggests that this may be wrong. On 6 September 1939, just three days after war broke out, two Oxford scientists applied for a grant to study penicillin, still thinking in terms of science, rather than application. They had been working on it for more than a year by now, and the outbreak of war actually made it harder for them to get the money. Both the Medical Research Council and the Rockefeller Foundation gave considerably less than requested, and the latter cited wartime uncertainty as the reason. So, if anything, the outbreak of war slowed down the development of penicillin at this stage.
The two scientists, Ernst Chain, a refugee biochemist from Germany, and Howard Florey, a pathologist from Australia, had come across Fleming’s work and decided to take a closer look before war began. Despite wartime shortages of suitable materials, money and people, by May 1940 their colleague Norman Heatley had extracted penicillin and injected it into mice to show that it did not harm them. On Saturday, 25 May, Florey injected four mice with penicillin before infecting them, and four control mice, with a huge dose of streptococcus bacteria. That night the four untreated mice died; the treated mice survived.
It dawned on Florey, Chain and Heatley that a new treatment for wounded soldiers might be at hand. Over the next few months they turned their laboratory into a penicillin factory, and on 12 February 1941 Albert Alexander, a 43-year-old policeman who was dying of septicaemia following a scratch from a rose bush, became the first person to be treated with penicillin. He quickly rallied, but supplies of penicillin dried up before he was fully cured, and he relapsed and sadly died. But the miraculous effect of the drug had been observed. Then in August 1942 Fleming (whose interest had now been reawakened) used penicillin to cure one Harry Lambert of meningitis, in a case that caught the attention of the press. From then on Fleming was a hero, more so than the publicity-shy Florey.
In July 1941, with the war stretching British industry to breaking point, Florey and Heatley flew to America to kick-start the production of penicillin there. Higher-yielding varieties of mould were quickly discovered and better techniques for culturing them, but chemical companies were initially reluctant to invest in such an uncertain project, while anti-trust (i.e. anti-monopoly) rules made it hard for the firms to learn techniques from each other. Britain was later somewhat resentful of just how much intellectual property relating to penicillin was then claimed by American industry.
Wartime shortages, security concerns and – in Britain – V1 flying bombs continued to hamper the project, so it is by no means clear that penicillin would have been developed more slowly in peacetime. This is not to deny the drug’s value to wounded soldiers, many of whose lives were saved. Even more remarkable was penicillin’s ability to cure gonorrhoea, an enemy that was causing more casualties than the Germans in the North African and Sicilian campaigns. By D-Day enough penicillin was available to ensure that the death rate from wounds was far lower than expected.
News of penicillin’s properties had reached Germany even before the war, and Hitler’s doctor used it to treat the Führer after the assassination attempt of June 1944, but no serious attempt was made to scale up production there or in France. This too would probably have been different in a peaceful 1940s.
The story of penicillin reinforces the lesson that even when a scientific discovery is made, by serendipitous good fortune, it takes a lot of practical work to turn it into a useful innovation.
The pursuit of polio
By the 1950s the most high-profile disease in the United States was polio. The story of polio’s vaccine is not quite as neat and harmless as that of smallpox. Some of the worries of the early opponents of Lady Mary Wortley Montagu did indeed come to pass, albeit much later. Vaccines did cause deaths that they should not have done. And it was another stubborn, unfashionable woman who blew the whistle.
Her name was Bernice Eddy. Born in rural West Virginia in 1903, the daughter of a doctor, she could not afford medical school, so she went into laboratory research, receiving a PhD in bacteriology in 1927 from the University of Cincinnati. By 1952 she was working on the polio virus in the Division of Biological Standards, a branch of the US government. She was involved in testing the new Salk vaccine for safety and efficacy.
Polio became a worsening epidemic especially in the United States during the twentieth century. Ironically, it was mainly improved public health that caused this, by raising the age at which most people caught the virus, resulting in more virulent infections and frequent paralysis. When everybody encountered sewage in their drinking or swimming water, the population was immunized early, before the virus caused paralysis. With chlorine cleaning up the water supply, people encountered the virus later and more virulently. By the 1950s the polio epidemic in the United States was worsening every year: 10,000 cases in 1940, 20,000 in 1945, 58,000 in 1952. Enormous public interest channelled generous donations into treatment, and the search for a vaccine. Huge fame and great wealth awaited the team that reached the prize, so some corners were cut.
One breakthrough came when Jonas Salk in Pittsburgh used the new technique of tissue culture to grow vast quantities of polio virus in the minced kidneys of monkeys. By 1953 he was killing fifty monkeys a week for their kidneys, growing the viruses in flasks of kidney tissue culture and inactivating them with thirteen days of exposure to formaldehyde. The vaccine thus produced was tested on 161 children and found to cause no harm, no polio and raised antibodies against the polio virus. Overcoming objections from Salk’s rivals, especially Albert Sabin, and ignoring the results of Bernice Eddy, who found that the vaccine could still sometimes cause polio in monkeys, the Salk vaccine was rushed into nationwide trials in 1955 with a great fanfare of publicity. Disaster followed when one of the manufacturers, Cutter Laboratories, infected thousands and paralysed more than 200 people with polio through inadequately inactivated virus. The vaccine was quickly withdrawn and the programme rethought.