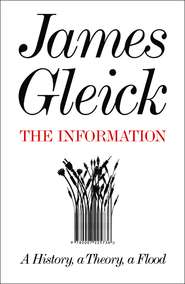
Полная версия:
The Information: A History, a Theory, a Flood
The English language no longer has such a thing as a geographic center, if it ever did. The universe of human discourse always has backwaters. The language spoken in one valley diverges from the language of the next valley, and so on. There are more valleys now than ever, even if the valleys are not so isolated. “We are listening to the language,” said Peter Gilliver, an OED lexicographer and resident historian. “When you are listening to the language by collecting pieces of paper, that’s fine, but now it’s as if we can hear everything said anywhere. Take an expatriate community living in a non-English-speaking part of the world, expatriates who live at Buenos Aires or something. Their English, the English that they speak to one another every day, is full of borrowings from local Spanish. And so they would regard those words as part of their idiolect, their personal vocabulary.” Only now they may also speak in chat rooms and on blogs. When they coin a word, anyone may hear. Then it may or may not become part of the language.
If there is an ultimate limit to the sensitivity of lexicographers’ ears, no one has yet found it. Spontaneous coinages can have an audience of one. They can be as ephemeral as atomic particles in a bubble chamber. But many neologisms require a level of shared cultural knowledge. Perhaps bada-bing would not truly have become part of twenty-first-century English had it not been for the common experience of viewers of a particular American television program (though it is not cited by the OED).
The whole word hoard—the lexis—constitutes a symbol set of the language. It is the fundamental symbol set, in one way: words are the first units of meaning any language recognizes. They are recognized universally. But in another way it is far from fundamental: as communication evolves, messages in a language can be broken down and composed and transmitted in much smaller sets of symbols: the alphabet; dots and dashes; drumbeats high and low. These symbol sets are discrete. The lexis is not. It is messier. It keeps on growing. Lexicography turns out to be a science poorly suited to exact measurement. English, the largest and most widely shared language, can be said very roughly to possess a number of units of meaning that approaches a million. Linguists have no special yardsticks of their own; when they try to quantify the pace of neologism, they tend to look to the dictionary for guidance, and even the best dictionary runs from that responsibility. The edges always blur. A clear line cannot be drawn between word and unword.
So we count as we can. Robert Cawdrey’s little book, making no pretense to completeness, contained a vocabulary of only 2,500. We possess now a more complete dictionary of English as it was circa 1600: the subset of the OED comprising words then current. That vocabulary numbers 60,000 and keeps growing, because the discovery of sixteenth-century sources never ends. Even so, it is a tiny fraction of the words used four centuries later. The explanation for this explosive growth, from 60,000 to a million, is not simple. Much of what now needs naming did not yet exist, of course. And much of what existed was not recognized. There was no call for transistor in 1600, nor nanobacterium, nor webcam, nor fen-phen. Some of the growth comes from mitosis. The guitar divides into the electric and the acoustic; other words divide in reflection of delicate nuances (as of March 2007 the OED assigned a new entry to prevert as a form of pervert, taking the view that prevert was not just an error but a deliberately humorous effect). Other new words appear without any corresponding innovation in the world of real things. They crystallize in the solvent of universal information.
What, in the world, is a mondegreen? It is a misheard lyric, as when, for example, the Christian hymn is heard as “Lead on, O kinky turtle . . .”). In sifting the evidence, the OED first cites a 1954 essay in Harper’s Magazine by Sylvia Wright: “What I shall hereafter call mondegreens, since no one else has thought up a word for them.” She explained the idea and the word this way:
When I was a child, my mother used to read aloud to me from Percy’s Reliques, and one of my favorite poems began, as I remember:
Ye Highlands and ye Lowlands,
Oh, where hae ye been?
They hae slain the Earl Amurray,
And Lady Mondegreen.
There the word lay, for some time. A quarter-century later, William Safire discussed the word in a column about language in The New York Times Magazine. Fifteen years after that, Steven Pinker, in his book The Language Instinct, offered a brace of examples, from “A girl with colitis goes by” to “Gladly the cross-eyed bear,” and observed, “The interesting thing about mondegreens is that the mishearings are generally less plausible than the intended lyrics.” But it was not books or magazines that gave the word its life; it was Internet sites, compiling mondegreens by the thousands. The OED recognized the word in June 2004.
A mondegreen is not a transistor, inherently modern. Its modernity is harder to explain. The ingredients—songs, words, and imperfect understanding—are all as old as civilization. Yet for mondegreens to arise in the culture, and for mondegreen to exist in the lexis, required something new: a modern level of linguistic self-consciousness and interconnectedness. People needed to mishear lyrics not just once, not just several times, but often enough to become aware of the mishearing as a thing worth discussing. They needed to have other such people with whom to share the recognition. Until the most modern times, mondegreens, like countless other cultural or psychological phenomena, simply did not need to be named. Songs themselves were not so common; not heard, anyway, on elevators and mobile phones. The word lyrics, meaning the words of a song, did not exist until the nineteenth century. The conditions for mondegreens took a long time to ripen. Similarly, the verb to gaslight now means “to manipulate a person by psychological means into questioning his or her own sanity”; it exists only because enough people saw the 1944 film of that title and could assume that their listeners had seen it, too. Might not the language Cawdrey spoke—which was, after all, the abounding and fertile language of Shakespeare—have found use for such a word? No matter: the technology for gaslight had not been invented. Nor had the technology for motion pictures.
The lexis is a measure of shared experience, which comes from inter-connectedness. The number of users of the language forms only the first part of the equation: jumping in four centuries from 5 million English speakers to a billion. The driving factor is the number of connections between and among those speakers. A mathematician might say that messaging grows not geometrically, but combinatorially, which is much, much faster. “I think of it as a saucepan under which the temperature has been turned up,” Gilliver said. “Any word, because of the interconnectedness of the English-speaking world, can spring from the backwater. And they are still backwaters, but they have this instant connection to ordinary, everyday discourse.” Like the printing press, the telegraph, and the telephone before it, the Internet is transforming the language simply by transmitting information differently. What makes cyberspace different from all previous information technologies is its intermixing of scales from the largest to the smallest without prejudice, broadcasting to the millions, narrowcasting to groups, instant messaging one to one.
This comes as quite an unexpected consequence of the invention of computing machinery. At first, that had seemed to be about numbers.
Chapter Four
To Throw the Powers of Thought into Wheel-Work
(Lo, the Raptured Arithmetician)
Light almost solar has been extracted from the refuse of fish; fire has been sifted by the lamp of Davy; and machinery has been taught arithmetic instead of poetry.
—Charles Babbage (1832)
NO ONE DOUBTED THAT Charles Babbage was brilliant. Nor did anyone quite understand the nature of his genius, which remained out of focus for a long time. What did he hope to achieve? For that matter, what, exactly, was his vocation? On his death in London in 1871 the Times obituarist declared him “one of the most active and original of original thinkers” but seemed to feel he was best known for his long, cranky crusade against street musicians and organ-grinders. He might not have minded. He was multifarious and took pride in it. “He showed great desire to inquire into the causes of things that astonish childish minds,” said an American eulogist. “He eviscerated toys to ascertain their manner of working.” Babbage did not quite belong in his time, which called itself the Steam Age or the Machine Age. He did revel in the uses of steam and machinery and considered himself a thoroughly modern man, but he also pursued an assortment of hobbies and obsessions—cipher cracking, lock picking, lighthouses, tree rings, the post—whose logic became clearer a century later. Examining the economics of the mail, he pursued a counterintuitive insight, that the significant cost comes not from the physical transport of paper packets but from their “verification”—the calculation of distances and the collection of correct fees—and thus he invented the modern idea of standardized postal rates. He loved boating, by which he meant not “the manual labor of rowing but the more intellectual art of sailing.” He was a train buff. He devised a railroad recording device that used inking pens to trace curves on sheets of paper a thousand feet long: a combination seismograph and speedometer, inscribing the history of a train’s velocity and all the bumps and shakes along the way.
As a young man, stopping at an inn in the north of England, he was amused to hear that his fellow travelers had been debating his trade:
“The tall gentleman in the corner,” said my informant, “maintained you were in the hardware line; whilst the fat gentleman who sat next to you at supper was quite sure that you were in the spirit trade. Another of the party declared that they were both mistaken: he said you were travelling for a great iron-master.”
“Well,” said I, “you, I presume, knew my vocation better than our friends.”
“Yes,” said my informant, “I knew perfectly well that you were in the Nottingham lace trade.”
He might have been described as a professional mathematician, yet here he was touring the country’s workshops and manufactories, trying to discover the state of the art in machine tools. He noted, “Those who enjoy leisure can scarcely find a more interesting and instructive pursuit than the examination of the workshops of their own country, which contain within them a rich mine of knowledge, too generally neglected by the wealthier classes.” He himself neglected no vein of knowledge. He did become expert on the manufacture of Nottingham lace; also the use of gunpowder in quarrying limestone; precision glass cutting with diamonds; and all known uses of machinery to produce power, save time, and communicate signals. He analyzed hydraulic presses, air pumps, gas meters, and screw cutters. By the end of his tour he knew as much as anyone in England about the making of pins. His knowledge was practical and methodical. He estimated that a pound of pins required the work of ten men and women for at least seven and a half hours, drawing wire, straightening wire, pointing the wire, twisting and cutting heads from the spiral coils, tinning or whitening, and finally papering. He computed the cost of each phase in millionths of a penny. And he noted that this process, when finally perfected, had reached its last days: an American had invented an automatic machine to accomplish the same task, faster.
Babbage invented his own machine, a great, gleaming engine of brass and pewter, comprising thousands of cranks and rotors, cogs and gearwheels, all tooled with the utmost precision. He spent his long life improving it, first in one and then in another incarnation, but all, mainly, in his mind. It never came to fruition anywhere else. It thus occupies an extreme and peculiar place in the annals of invention: a failure, and also one of humanity’s grandest intellectual achievements. It failed on a colossal scale, as a scientific-industrial project “at the expense of the nation, to be held as national property,” financed by the Treasury for almost twenty years, beginning in 1823 with a Parliamentary appropriation of £1,500 and ending in 1842, when the prime minister shut it down. Later, Babbage’s engine was forgotten. It vanished from the lineage of invention. Later still, however, it was rediscovered, and it became influential in retrospect, to shine as a beacon from the past.
Like the looms, forges, naileries, and glassworks he studied in his travels across northern England, Babbage’s machine was designed to manufacture vast quantities of a certain commodity. The commodity was numbers. The engine opened a channel from the corporeal world of matter to a world of pure abstraction. The engine consumed no raw materials—input and output being weightless—but needed a considerable force to turn the gears. All that wheel-work would fill a room and weigh several tons. Producing numbers, as Babbage conceived it, required a degree of mechanical complexity at the very limit of available technology. Pins were easy, compared with numbers.
It was not natural to think of numbers as a manufactured commodity. They existed in the mind, or in ideal abstraction, in their perfect infinitude. No machine could add to the world’s supply. The numbers produced by Babbage’s engine were meant to be those with significance: numbers with a meaning. For example, 2.096910013 has a meaning, as the logarithm of 125. (Whether every number has a meaning would be a conundrum for the next century.) The meaning of a number could be expressed as a relationship to other numbers, or as the answer to a certain question of arithmetic. Babbage himself did not speak in terms of meaning; he tried to explain his engine pragmatically, in terms of putting numbers into the machine and seeing other numbers come out, or, a bit more fancifully, in terms of posing questions to the machine and expecting an answer. Either way, he had trouble getting the point across. He grumbled:
On two occasions I have been asked,—“Pray, Mr. Babbage, if you put into the machine wrong figures, will the right answers come out?” In one case a member of the Upper, and in the other a member of the Lower, House put this question. I am not able rightly to apprehend the kind of confusion of ideas that could provoke such a question.
Anyway, the machine was not meant to be a sort of oracle, to be consulted by individuals who would travel from far and wide for mathematical answers. The engine’s chief mission was to print out numbers en masse. For portability, the facts of arithmetic could be expressed in tables and bound in books.
To Babbage the world seemed made of such facts. They were the “constants of Nature and Art.” He collected them everywhere. He compiled a Table of Constants of the Class Mammalia: wherever he went he timed the breaths and heartbeats of pigs and cows. He invented a statistical methodology with tables of life expectancy for the somewhat shady business of life insurance. He drew up a table of the weight in Troy grains per square yard of various fabrics: cambric, calico, nankeen, muslins, silk gauze, and “caterpillar veils.” Another table revealed the relative frequencies of all the double-letter combinations in English, French, Italian, German, and Latin. He researched, computed, and published a Table of the Relative Frequency of the Causes of Breaking of Plate Glass Windows, distinguishing 464 different causes, no less than fourteen of which involved “drunken men, women, or boys.” But the tables closest to his heart were the purest: tables of numbers and only numbers, marching neatly across and down the pages in stately rows and columns, patterns for abstract appreciation.
A book of numbers: amid all the species of information technology, how peculiar and powerful an object this is. “Lo! the raptured arithmetician!” wrote Élie de Joncourt in 1762. “Easily satisfied, he asks no Brussels lace, nor a coach and six.” Joncourt’s own contribution was a small quarto volume registering the first 19,999 triangular numbers. It was a treasure box of exactitude, perfection, and close reckoning. These numbers were so simple, just the sums of the first n whole numbers: 1, 3 (1+2), 6 (1+2+3), 10 (1+2+3+4), 15, 21, 28, and so on. They had interested number theorists since Pythagoras. They offered little in the way of utility, but Joncourt rhapsodized about his pleasure in compiling them and Babbage quoted him with heartfelt sympathy: “Numbers have many charms, unseen by vulgar eyes, and only discovered to the unwearied and respectful sons of Art. Sweet joy may arise from such contemplations.”
Tables of numbers had been part of the book business even before the beginning of the print era. Working in Baghdad in the ninth century, Abu Abdullah Mohammad Ibn Musa al-Khwarizmi, whose name survives in the word algorithm, devised tables of trigonometric functions that spread west across Europe and east to China, made by hand and copied by hand, for hundreds of years. Printing brought number tables into their own: they were a natural first application for the mass production of data in the raw. For people in need of arithmetic, multiplication tables covered more and more territory: 10 × 1,000, then 10 × 10,000, and later as far as 1,000 × 1,000. There were tables of squares and cubes, roots and reciprocals. An early form of table was the ephemeris or almanac, listing positions of the sun, moon, and planets for sky-gazers. Tradespeople found uses for number books. In 1582 Simon Stevin produced Tafelen van Interest, a compendium of interest tables for bankers and moneylenders. He promoted the new decimal arithmetic “to astrologers, land-measurers, measurers of tapestry and wine casks and stereometricians, in general, mint masters and merchants all.” He might have added sailors. When Christopher Columbus set off for the Indies, he carried as an aid to navigation a book of tables by Regiomontanus printed in Nuremberg two decades after the invention of moveable type in Europe.
Joncourt’s book of triangular numbers was purer than any of these— which is also to say useless. Any arbitrary triangular number can be found (or made) by an algorithm: multiply n by n + 1 and divide by 2. So Joncourt’s whole compendium, as a bundle of information to be stored and transmitted, collapses in a puff to a one-line formula. The formula contains all the information. With it, anyone capable of simple multiplication (not many were) could generate any triangular number on demand. Joncourt knew this. Still he and his publisher, M. Husson, at the Hague, found it worthwhile to set the tables in metal type, three pairs of columns to a page, each pair listing thirty natural numbers alongside their corresponding triangular numbers, from 1(1) to 19,999(199,990,000), every numeral chosen individually by the compositor from his cases of metal type and lined up in a galley frame and wedged into an iron chase to be placed upon the press.
Why? Besides the obsession and the ebullience, the creators of number tables had a sense of their economic worth. Consciously or not, they reckoned the price of these special data by weighing the difficulty of computing them versus looking them up in a book. Precomputation plus data storage plus data transmission usually came out cheaper than ad hoc computation. “Computers” and “calculators” existed: they were people with special skills, and all in all, computing was costly.
Beginning in 1767, England’s Board of Longitude ordered published a yearly Nautical Almanac, with position tables for the sun, moon, stars, planets, and moons of Jupiter. Over the next half century a network of computers did the work—thirty-four men and one woman, Mary Edwards of Ludlow, Shropshire, all working from their homes. Their painstaking labor paid £70 a year. Computing was a cottage industry. Some mathematical sense was required but no particular genius; rules were laid out in steps for each type of calculation. In any case the computers, being human, made errors, so the same work was often farmed out twice for the sake of redundancy. (Unfortunately, being human, computers were sometimes caught saving themselves labor by copying from one other.) To manage the information flow the project employed a Comparer of the Ephemeris and Corrector of the Proofs. Communication between the computers and comparer went by post, men on foot or on horseback, a few days per message.
A seventeenth-century invention had catalyzed the whole enterprise. This invention was itself a species of number, given the name logarithm. It was number as tool. Henry Briggs explained:
Logarithmes are Numbers invented for the more easie working of questions in Arithmetike and Geometrie. The name is derived of Logos, which signifies Reason, and Arithmos, signifying Numbers. By them all troublesome Multiplications and Divisions in Arithmetike are avoided, and performed onely by Addition in stead of Multiplication, and by Subtraction in stead of Division.
In 1614 Briggs was a professor of geometry—the first professor of geometry—at Gresham College, London, later to be the birthplace of the Royal Society. Without logarithms he had already created two books of tables, A Table to find the Height of the Pole, the Magnetic Declination being given and Tables for the Improvement of Navigation, when a book came from Edinburgh promising to “take away all the difficultie that heretofore hath beene in mathematical calculations.”
There is nothing (right well beloved Students in the Mathematickes) that is so troublesome to Mathematicall practice, not that doth more molest and hinder Calculators, then the Multiplications, Divisions, square and cubical Extractions of great numbers, which besides the tedious expence of time, are for the most part subject to many slippery errors.
This new book proposed a method that would do away with most of the expense and the errors. It was like an electric flashlight sent to a lightless world. The author was a wealthy Scotsman, John Napier (or Napper, Nepair, Naper, or Neper), the eighth laird of Merchiston Castle, a theologian and well-known astrologer who also made a hobby of mathematics. Briggs was agog. “Naper, lord of Markinston, hath set my head and hands a work,” he wrote. “I hope to see him this summer, if it please God, for I never saw book, which pleased me better, and made me more wonder.” He made his pilgrimage to Scotland and their first meeting, as he reported later, began with a quarter hour of silence: “spent, each beholding other almost with admiration before one word was spoke.”
Briggs broke the trance: “My Lord, I have undertaken this long journey purposely to see your person, and to know by what engine of wit or ingenuity you came first to think of this most excellent help unto astronomy, viz. the Logarithms; but, my Lord, being by you found out, I wonder nobody else found it out before, when now known it is so easy.” He stayed with the laird for several weeks, studying.
In modern terms a logarithm is an exponent. A student learns that the logarithm of 100, using 10 as the base, is 2, because 100 = 102. The logarithm of 1,000,000 is 6, because 6 is the exponent in the expression 1,000,000 = 106. To multiply two numbers, a calculator could just look up their logarithms and add those. For example:
100 × 1,000,000 = 102 × 106 = 10(2 + 6)
Looking up and adding are easier than multiplying.
But Napier did not express his idea this way, in terms of exponents. He grasped the thing viscerally: he was thinking in terms of a relationship between differences and ratios. A series of numbers with a fixed difference is an arithmetic progression: 0, 1, 2, 3, 4, 5 . . . When the numbers are separated by a fixed ratio, the progression is geometric: 1, 2, 4, 8, 16, 32 . . . Set these progressions side by side,