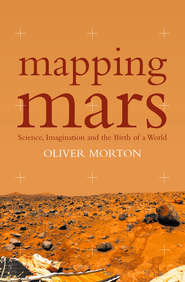
Полная версия:
Mapping Mars: Science, Imagination and the Birth of a World

OLIVER MORTON
Mapping Mars
SCIENCE, IMAGINATION AND
THE BIRTH OF A WORLD
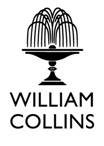
Copyright
William Collins
An imprint of HarperCollinsPublishers 77–85 Fulham Palace Road, London W6 8JB www.harpercollins.co.uk
First published in Great Britain by William Collins in 2002
Copyright © Abq72 Ltd 2002
The right of Oliver Morton to be identified as the author of this work has been asserted by him in accordance with the Copyright, Designs and Patents Act 1988
A catalogue record for this book is available from the British Library
All rights reserved under International and Pan-American Copyright Conventions. By payment of the required fees, you have been granted the nonexclusive, nontransferable right to access and read the text of this e-book on-screen. No part of this text may be reproduced, transmitted, downloaded, decompiled, reverse engineered, or stored in or introduced into any information storage and retrieval system, in any form or by any means, whether electronic or mechanical, now known or hereinafter invented, without the express written permission of HarperCollins e-books.
HarperCollinsPublishers has made every reasonable effort to ensure that any picture content and written content in this ebook has been included or removed in accordance with the contractual and technological constraints in operation at the time of publication.
Source ISBN: 9781841156699
Ebook Edition © JUNE 2012 ISBN: 9780007397051
Version: 2014-09-15
Dedication
For Lieutenant Arthur Noel Morton, RNVR
Navigating officer, HMS Hargood, 1944–5 Lover of maps, lover of writing and loving father – ‘Round about here, Sir.’
Contents
Cover
Title Page
Copyright
Dedication
Preface
Introduction
Part One – Maps
Greenwich
A Point of Warlike Light
Mert Davies’s Net
The Polar Lander
Mariner 9
The Art of Drawing
The Laser Altimeter
Part Two – Histories
Meteor Crater
‘A Little Daft on the Subject of the Moon’
An Antique Land
Maps and Multiple Hypotheses
The Artist’s Eye
Layers
Part Three – Water
Malham
Mike Carr’s Mars
Reflections
Shorelines
The Ocean Below
‘Common Sense and Uncommon Subtlety’
Part Four – Places
Buffalo
Putting Together a Place
The Underground
Bob Zubrin’s Frontier
Mapping Martians
Part Five – Change
Symbols of the Future
Gaia’s Neighbour
The Undiscover’d Country
Bibliography
Index
Acknowledgements
About the Author
References, Notes and Further Reading
About the Publisher
Preface
‘Are you going to move our stuff?’
‘No, that’s the view. We’re in the picture.’
Exchange between William Fox and Mark Klett
in William L. Fox, View Finder
Introduction
There’s a world on my wall.
Mountains, canyons, plains and valleys, all a faded pinkish ochre, an even tone as plain as a colour can be without being grey. The sun is to the west – shadows fall gently to the right. There are faults and rifts, ash flows and lava fields. There are creases and stretch marks, straight lines and strange curves. There are circles and circles and circles.
No cities. No seas. No forests and no battlegrounds. No prairies. No nations. No histories and no legends. No memories. Just features, features and names. Argyre and Hellas and Isidis. Olympus and Alba and Pavonis. Schiaparelli and Antoniadi, Kasei and Nirgal. Beautiful double-rimmed Lowell. Names from one world projected on to maps of another. Maps of Mars.
The maps on my wall, painstakingly painted about fifteen years ago, show the surface of Mars from pole to pole. They show volcanoes that dwarf their earthly cousins in age and size. They show the round scars of uncountable asteroid impacts, many far more violent than the one which killed off the earth’s dinosaurs. They show a canyon so long and deep it’s as if the planet’s tight skin has swollen and split. They show featureless plains and pock-marked ones, jumbled hummocky hills and strange creases that swarm together for thousands of kilometres, like the grain in a piece of timber. They show features perfectly earthlike and features so strange the earth has no names for them. There’s a world’s worth of scientific puzzles here, some of them already tentatively answered, most still mysterious. There’s a world’s worth of possibilities. But there’s no clear place to start the story.
If people had moved across the pinkish ochre – if they had grown vines on the terraces of Olympus, or herded goats through the Labyrinths of the Night; if legends haunted Tempe and the dales of Arcadia, or if in Ares Vallis ancient grudge had broken into new mutiny – then it would be easy. But there are none of those tales to tell. No gardens of Eden, no sacred springs, nowhere to start the story of a world.
Even stripped of people, with their cities and their borders and their histories, a map of earth would not be this unyielding. Global truths and discrete units of geography would draw the eye. River catchments would tile the plains, mountain ranges would stand like the backbones of continents. There would be seas and islands, well defined. But Mars is not like that. It is continuous, seamless and sealess. Its great mountains stand alone; there are no sweeping ranges, no Rockies or Alps or Andes. The rivers are long gone. There are no continents and there are no oceans, and thus there are no shores. Given patience, provisions and a pressure suit you could walk from any point on the planet to any other. No edges guide the eye or frame the scene. Nowhere says: Start Here.
We might begin the story at one of the places that humanity has touched. In 1971 a Russian spacecraft crashed into Hellas, a vast basin in the southern hemisphere, while another landed more decorously on the other side of the planet, somewhere in or around the crater Ptolemaus. Two years later another Russian probe struck the surface somewhere near the dry valley called Samara. None sent back anything by way of a message. In 1976 America’s more sophisticated Viking landers lowered themselves gently to sites in the northern plains of Chryse and utopia, sending back panoramas of rock and rubble beneath pink-looking skies. But the Vikings eventually fell silent too, leaving Mars alone again. Preludes, not beginnings.
Twenty years later, NASA’s little Pathfinder, cocooned in airbags, bounced to a halt in the rocky fields where Ares Vallis had once spewed out its flood waters. It let loose Sojourner, the first of humanity’s creations to travel on its own across the sands of Mars. That was a new beginning, the beginning of a grand age for earth’s robots. At the time of writing there have been automatic envoys sending data back from Mars ever since. But Pathfinder’s story cannot encompass the whole vast world in front of me. Not yet.
What about beginning on earth? Some places here are very like locations there, perhaps close enough to be tied together by some sort of sympathetic story-magic. Maybe Antarctica, where the driest, coldest landscapes on earth are regularly visited by scientists wanting to get some sense of a smaller, drier, colder world. Or Iceland, where permafrost and lava fight as once they did on Mars. Or the scablands of Washington state, ripped clean by floods like those that scoured Pathfinder’s landing site. Or Hawaii’s volcanoes, near perfect miniatures of the Martian giants. Or Arizona’s Meteor Crater, where earthly geologists first came to grips with what a little bit of asteroid can do to the face of a planet, given enough speed. They are all places where one can learn about Mars, where the trained imagination can almost touch it. But none evokes the whole world.
We could cast our imaginations wider, to those who have tried to speak for all of Mars. To the astronomers looking at it with their telescopes, measuring all the qualities of light reflected from its surface, seeing seasons and imagining civilisations. Or to the writers inspired by those astronomical visions: H. G. Wells and Stanley Weinbaum, Arthur C. Clarke and Robert Heinlein, Ray Bradbury and Alexander Bogdanov and Edgar Rice Burroughs. Their imaginations took a point of light and turned it into a world of experience. But their Mars was never this one, the one which we only saw – which we could only ever see – after our envoys left the earth and went there.
Only after our spacecraft reached its orbit could we see Mars for what it is, a planet with a surface area as great as that of the earth’s continents, all of it as measurable, as real as the stones in the pavement outside your door. After millennia of talking about worlds beyond our own, of heavens and hells and the Isles of the Hesperides, humanity now has such a world fixed in its sights, solid and sure. For the moment it is a world of science, untouchable but inspectable and oddly accessible, if only through the most complex of tools. But unlike the other worlds that scientists create with their imaginations and instruments – the worlds of molecular dynamics and of inflationary cosmology and all the rest of them – this one is on the edge of being a world in the oldest, truest, sense. A world of places and views, a world that would graze your knees if you fell on it, a world with winds and sunsets and the palest of moonlight. Almost a world like ours, except for the emptiness.
This book is about how ideas from our full and complex planet are projected on to the rocks of that simpler, empty one. The ideas discussed are mostly scientific, because it is the scientists who have thought hardest and best about the realities of Mars. It is the scientists who have fathomed the ages of its rocks, measured its resemblance to the earth, searched for its missing waters and – always – wondered about the life it might be home to. The stories they tell about the planet must have pride of place. But there are artists in here too, and writers, and poets, and people whose dreams take no such articulated form, but still focus themselves on the same rocks in the sky. They illuminate Mars; Mars illuminates them.
It’s common to imagine that the human story on Mars will only start when humans actually get there, when they stand beneath its dusty sky and look around them at its oddly close horizon. I don’t know who those people will be, or when they will get there, or where on the planet they will first set their feet. But I know that for all their importance, they will not be a new story’s beginning, rather a new chapter. Their expectations and hopes are already being created on the earth today, by the people in this book; the process of making Mars into a human world has already begun. And I know that their landing site is somewhere on the map in front of me, already charted, if not yet chosen.
Back to the maps, then; in particular to the 1:15,000,000 shaded-relief map of the surface published by the United States Geological Survey, its three sheets fixed to my office wall. It represents the planet as well as any single image could. But it’s not just the representation of a planet. It’s the embodiment of a process, a process that forged links between far-off Mars and the cartographers’ drawing board point by point, feature by feature. It embodies links of reason and technology that ran through the cameras of now-dead spacecraft millions of kilometres away, and through the minds of the men who designed and controlled those cameras. Links that ran through empty space, carried by the faintest of radio waves, and through the great dishes that picked up those signals, and through the computers that wove them back into images. Links that ran through the eyes and minds and hands of the people who assembled the pictures produced by that great scientific adventure into a world they could see in their minds and draw on the paper in front of them, a world precise and publishable.
The maps themselves tell no single story. But the people who put those links together with technology and craft, mathematics and imagination – they have a story, one that lets the maps and the planet they are tied to come to life.
Where to begin to write about Mars? With the making of the maps.
Part I – Maps
‘Now when I was a little chap I had a passion for maps. I would look for hours at South America, or Africa, or Australia, and lose myself in all the glories of exploration. At that time there were many blank spaces on the earth, and when I saw one that looked particularly inviting on a map (but they all look that) I would put my finger on it and say, “When I grow up I will go there.” The North Pole was one of these places, I remember. Well, I haven’t been there yet, and shall not try now. The glamour’s off. Other places were scattered about the Equator, and in every sort of latitude all over the two hemispheres. I have been in some of them, and … well, we won’t talk about that. But there was one yet – the biggest, the most blank, so to speak – that I had a hankering after …’
Marlow in Joseph Conrad, Heart of Darkness
Greenwich
And then, as they sat looking at the ships and steamboats making their way to the sea with the tide that was running down, the lovely woman imagined all sorts of voyages for herself and Pa.
Charles Dickens, Our Mutual Friend
Maps of the earth begin a short walk from the flat where I live. Go down the High Road, up Royal Hill towards the butcher’s, left along Burney Street and then right on to Crooms Hill. At the corner, if you care for such things, you can see a blue plaque of the sort with which London marks houses where people who have made a significant contribution to human happiness once lived. In this case, it was the poet Cecil Day Lewis; as you climb the hill, you’ll pass another one marking the home of Benjamin Waugh, founder of the National Society for the Prevention of Cruelty to Children.
Near the top of the hill sits a grand (but plaqueless) bow-fronted white house, called simply the White House. Walk round the White House’s walled garden, down a little alleyway and through a gate in the high brick wall on your right, and you emerge into Greenwich Park. To your right, the beautiful semicircle of the rose garden; to your left a steep path lined by trees. And as you walk out on to the grass, London spread at your feet. As views go, it’s not particularly extensive – the horizon is nowhere more than twenty kilometres away and in many directions much closer – but it’s vast in association. The once imperial cityscape is woven from threads that stretch throughout the world.
Across the river to the east sits the squat black-glass bulk of Reuters, information from around the globe splashing into its rooftop dishes. Upstream and on the near side sit the long, low workshops where for more than a century men have made undersea cables to tie the continents together. New skyscrapers devoted to global businesses sit in the redeveloped heart of the docks that used to handle the lion’s share of the world’s sea trade. Within the park itself there are plants from every continent except Antarctica. At its foot sits the old naval college, where generations of Britannia’s officers, my late father included, learned to rule the waves.
Through it all the Thames runs softly, looping around the Isle of Dogs, a local feature leading, as Conrad says in Heart of Darkness, ‘to the uttermost ends of the earth’. Little sails down this umbilicus of empire now – but above it the new trade routes of the sky are sketched out by aircraft arriving and departing from London’s four airports, carving their way through the air we all breathe and the stratosphere we shelter under. To the west the Thames beneath them is still daytime blue; to the east it is already evening dark.
Dawn may feel like an intervention by the sun, rising above a stationary earth; sunset reveals the truth of the earth’s turning, a slipping away into night. That turning defines two unique, unmoving points on the surface of the earth: the poles, the extremes of latitude. Add one more point – just one – and you have a co-ordinate system that can describe the whole world, a basis for all the maps and charts the sailors and pilots need, a way of deciding when days start and end. And that third point is right in front of you, the strongest of all Greenwich’s links to the rest of the earth. In the middle of the park is the old Royal Observatory, a little gathering of domes perched clubbily on a ridge. Within the observatory sits a massive metal construction called a transit circle. The line passing through the poles and through that transit circle is the earth’s prime meridian: 0 degrees, 0 minutes, 0 seconds. All earthly longitudes are measured with respect to that line through Greenwich Park.
The English have taken the Greenwich meridian as the starting point for longitudes since the observatory was founded in the seventeenth century. But it wasn’t until the late nineteenth century – at a time when its home in Greenwich was under the stewardship of Sir George Airy, Astronomer Royal, the man who had that great transit circle built – that the Greenwich meridian was formally adopted by the rest of the world. With worldwide navigation a commonplace, and with telecommunications making almost instantaneous contact between continents a possibility, there was a need for a single set of co-ordinates to define the world’s places and time zones. Over the years a variety of possible markers to define this prime meridian were suggested – islands, mountains, artefacts like the Great Pyramid or the Temple in Jerusalem. But a meridian defined by an observatory seemed best. In 1884, at a conference in Washington DC, and over spirited French opposition, Greenwich was chosen. Airy’s transit circle came to define the world.
Airy was, by all accounts, an uninspiring but meticulous man. He recorded his every thought and expenditure from the day he went up to Cambridge University to more or less the day he died, throwing no note away, delighting in doing his own double-entry bookkeeping. He applied a similar thoroughness to his stewardship over the Royal Greenwich Observatory, bringing to its workings little interest in theory or discovery but a profound concern for order, which meant that the production of tables for the Admiralty (the core of the observatory’s job) was accomplished with mechanical accuracy. He looked at the heavens and the earth with precision, not wonder, and though he had his fancies, they were fancies in a similar vein – ecstasies of exactitude such as calculating the date of the Roman invasion of Britain from Caesar’s account of the timing of the tides, or meticulously celebrating the geographical accuracy of Sir Walter Scott’s poem ‘The Lady of the Lake’. This was a man whose love of a world where everything was in its place would lead him to devote his own time to sticking labels saying ‘empty’ on empty boxes rather than disturb the smooth efficiency of the observatory by taking an underling from his allotted labours to do so for him. After more than forty-five years of such service Airy eventually retired 200 yards across the park to the White House on Crooms Hill, where he died a decade later.
It’s a little sad that the White House doesn’t carry a blue circular plaque to commemorate Airy’s part in the happiness brought to humanity by a single agreed-upon meridian, but surely there are monuments elsewhere. Maybe Ipswich has an Airy Street; he grew up there and remained fond of the place, arranging for his great transit circle to be made at an Ipswich workshop. There must be a bust of him in the Royal Astronomical Society. Or a portrait in some Cambridge common room. And even if there are none of these things, there is something far grander. Wherever else astronomers go when they die, those who have shown even the faintest interest in the place are welcomed on to the planet Mars, at least in name. By international agreement, craters on Mars are named after people who have studied the planet or evoked it in their creative work – which mostly makes Mars a mausoleum for astronomers, with a few science fiction writers thrown in for spice. In the decades since the craters of Mars were first discovered by space probes, hundreds of astronomers have been thus immortalised. But none of them has a crater more fitting than Airy’s.
A Point of Warlike Light
‘I’ve never been to Mars, but I imagine it to be quite lovely.’
Cosmo Kramer, in Seinfeld
(‘The Pilot (I)’, written by Larry David)
Mars had an internationally agreed prime meridian before the earth did. In 1830 the German astronomers Wilhelm Beer and Johann von Mädler, famous now mostly for their maps of the moon, turned their telescope in Berlin’s Tiergarten to Mars. The planet had been observed before. Its polar caps were known, and so was its changeability; the face of Mars varies from minute to minute, due to the earth’s distorting atmosphere, and from season to season, due to quite different atmospheric effects on Mars itself. There are, though, some features that can be counted on to stick around from minute to minute and season to season, the most notable being the dark region now called Syrtis Major, then known as the Hourglass Sea. To calculate the length of the Martian day, Mädler (Beer owned the telescope – Mädler did most of the work) chose another, smaller dark region, precisely timing its reappearance night after night. He got a figure of 24 hours 37 minutes and 9.9 seconds, 12.76 seconds less than the currently accepted figure. That this length of time is so similar to the length of an earthly day is complete coincidence, one of three coincidental similarities between the earth and Mars. The second coincidence is that the obliquity of Mars – the angle that its axis of rotation makes with a notional line perpendicular to the plane of its orbit – is, at 25.2°, very similar to the obliquity of the earth. The third is that though Mars is considerably smaller than the earth – a little more than half its radius, a little more than a tenth its mass – its surface area, at roughly a third of the earth’s, is quite similar to that of the earth’s continents.
When Mädler came to compile his observations into a chart in 1840, mathematically transforming his sketches of the disc of Mars into a rectangular Mercator projection, he declined to name the features he recorded, but did single out the small dark region he had used to time the Martian day as the site of his prime meridian, centring his map on it. Future astronomers followed him in the matter of the meridian while eagerly making good his oversight in the matter of names. Father Angelo Secchi, a Jesuit at the Vatican observatory, turned the light and dark patches into continents and seas, respectively, as astronomers had done for the moon, and gave the resulting geographic features the names of famous explorers – save for the Hourglass Sea, which he renamed the ‘Atlantic Canale’, seeing it as a division between Mars’s old world and its new. In 1867 Richard Proctor, an Englishman who wrote popular astronomy books, produced a nomenclature based on astronomers, rather than explorers, and gave astronomers associated with Mars pride of place. His map has a Mädler Land and a Beer Sea, along with a Secchi Continent. Observations made by the Astronomer Royal in the 1840s – he was interested in making more precise measurements of the planet’s diameter – were commemorated by the Airy Sea. Pride of place went to the Rev. William Rutter Dawes, a Mars observer of ferociously keen eyesight, perceiving, for example, that the dark patch Mädler had used to mark the prime meridian had two prongs. (Dawes’s far-field acuity was allegedly compensated by a visual deficit closer to home; it is said he could pass his wife in the street without recognising her.) So great was Dawes’s influence on Proctor – or so small was the number of astronomers associated with Mars – that his name was given not just to the biggest ocean but also to a Continent, a Sea, a Strait, an Isle and, marking the meridian, his very own Forked Bay.