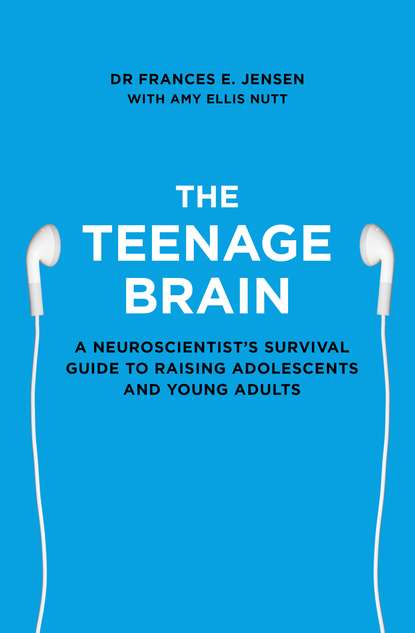
Полная версия:
The Teenage Brain: A neuroscientist’s survival guide to raising adolescents and young adults
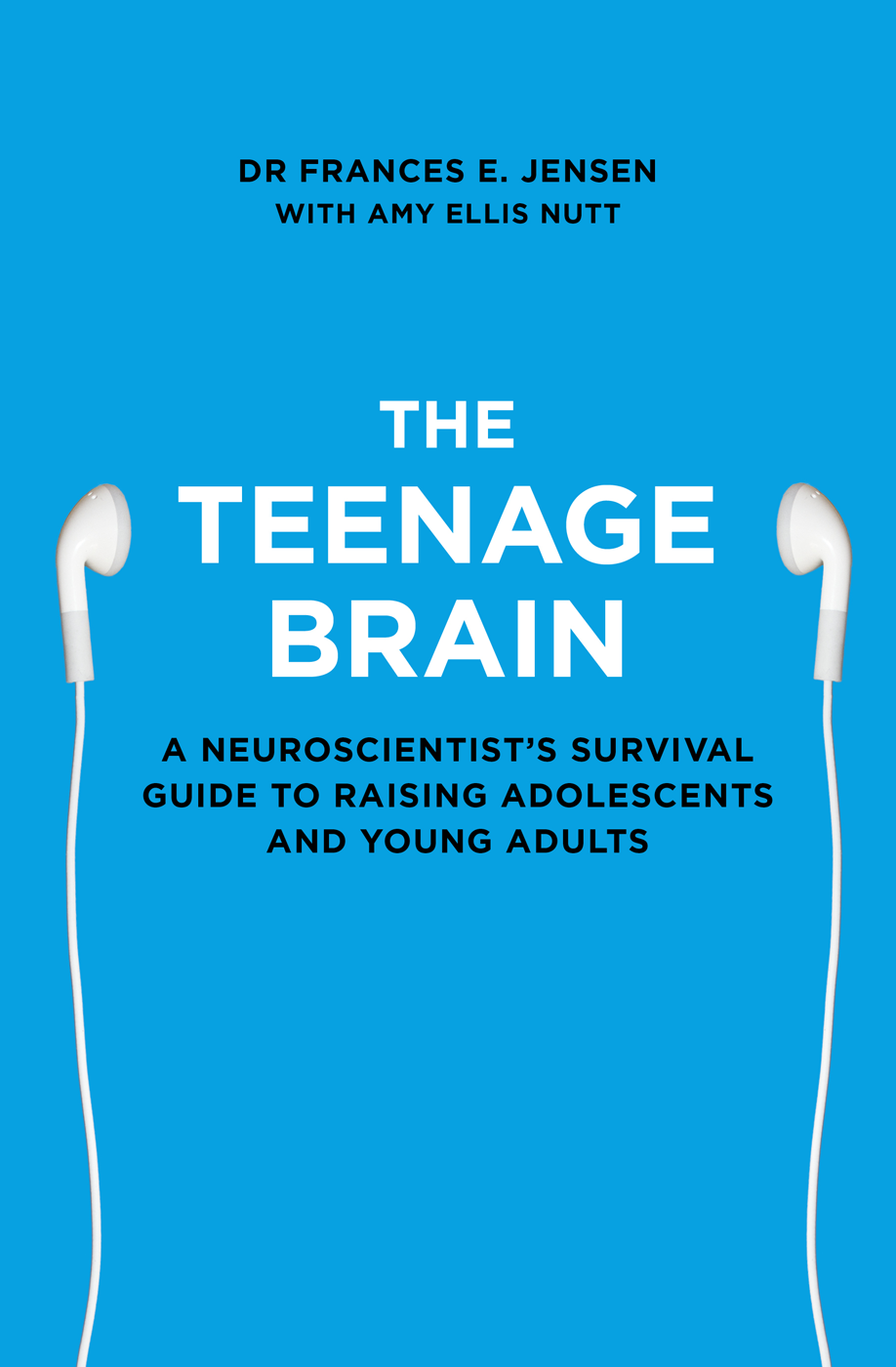
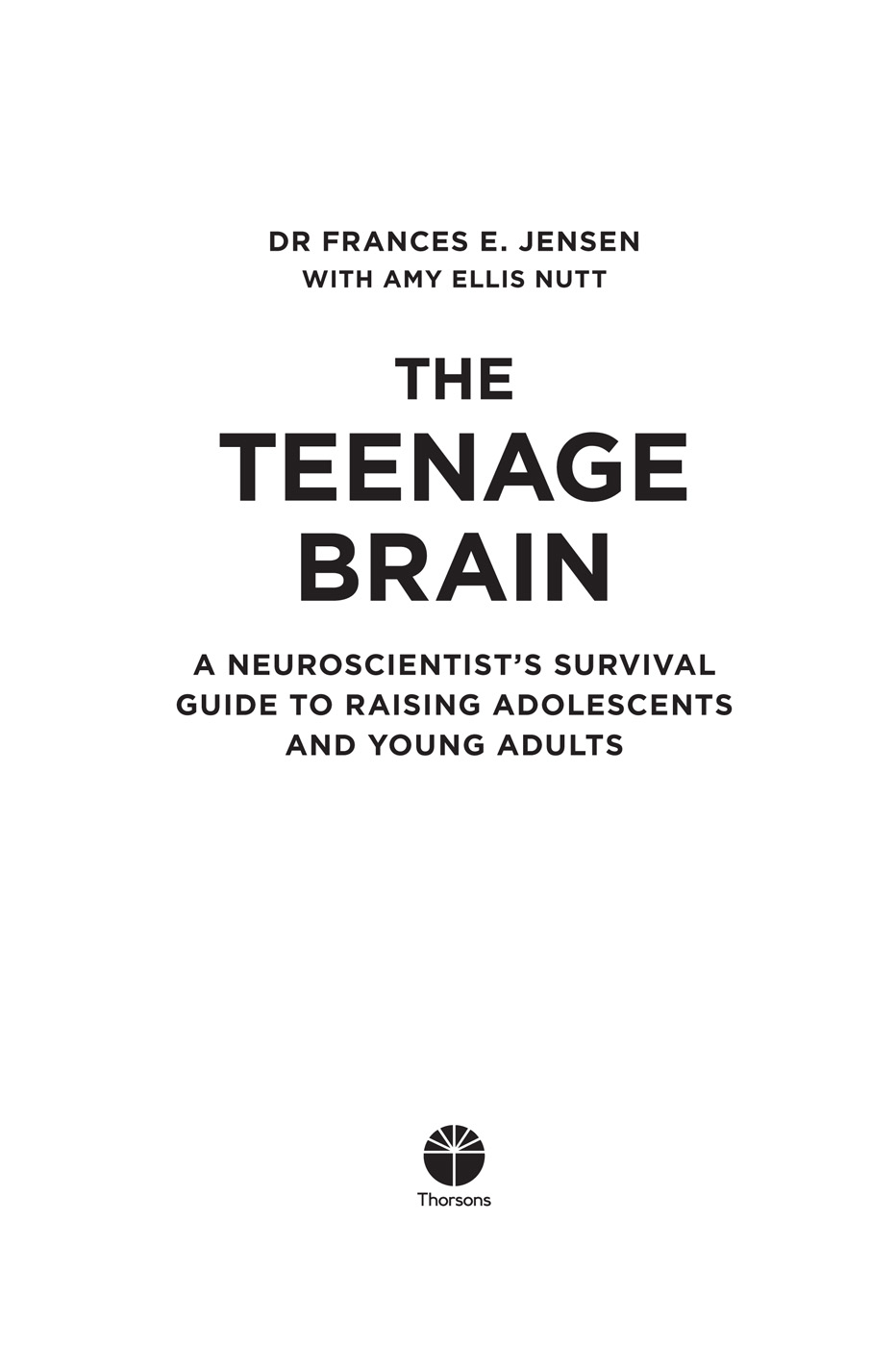
Copyright
This book is designed to give information on various medical conditions, treatments, and procedures for your personal knowledge and to help you be a more informed consumer of medical and health services. It is not intended to be complete or exhaustive, nor is it a substitute for the advice of your doctor. You should seek medical care promptly for any specific medical condition or problem you may have. All efforts have been made to ensure the accuracy of the information contained in this book as of the date published. The authors and the publisher expressly disclaim responsibility for any adverse effects arising from the use or application of the information contained herein.
HarperThorsons
An imprint of HarperCollinsPublisher 1 London Bridge Street London SE1 9GF
www.harpercollins.co.uk
First published in the US by HarperCollinsPublishers 2015 The edition published by HarperThorsons 2015
Designed by Jo Anne Metsch
© Frances E. Jensen with Amy Ellis Nutt 2015
The authors assert the moral right to be identified as the authors of this work
A catalogue record of this book is available from the British Library
All rights reserved under International and Pan-American Copyright Conventions. By payment of the required fees, you have been granted the nonexclusive, non-transferable right to access and read the text of this e-book on screen. No part of this text may be reproduced, transmitted, downloaded, decompiled, reverse engineered, or stored in or introduced into any information storage retrieval system, in any form or by any means, whether electronic or mechanical, now known or hereinafter invented, without the express written permission of HarperCollins e-books.
Find out more about HarperCollins and the environment at www.harpercollins.co.uk/green
Source ISBN: 9780007448319
Ebook Edition © December 2014 ISBN: 9780007448326
Version: 2014-12-15
This book is dedicated to my two sons, Andrew and Will. Watching them grow into young men as they emerged through their teen years has been the joy of my life, and shepherding them through this time was probably the most important job of my life. Together we went on a journey, and as much as I taught them, they taught me. The product is this book, and I hope that it informs not only those people helping to raise adolescents, but also the teenagers themselves.
When I was a boy of fourteen, my father was so ignorant I could hardly stand to have the old man around. But when I got to be twenty-one, I was astonished by how much he’d learned in seven years.
—MARK TWAIN
I would that there were no age between sixteen and three-and-twenty, or that youth would sleep out the rest, for there is nothing in the between but getting wenches with child, wronging the ancientry, stealing, fighting …
—THE WINTER’S TALE, WILLIAM SHAKESPEARE
Contents
Cover
Title Page
Copyright
Dedication
Epigraph
List of Illustrations
Introduction: Being Teen
1 Entering the Teen Years
2 Building a Brain
3 Under the Microscope
4 Learning: A Job for the Teen Brain
5 Sleep
6 Taking Risks
7 Tobacco
8 Alcohol
9 Pot
10 Hard-Core Drugs
11 Stress
12 Mental Illness
13 The Digital Invasion of the Teenage Brain
14 Gender Matters
15 Sports and Concussions
16 Crime and Punishment
17 Beyond Adolescence: It’s Not Over Yet
Postscript: Final Thoughts
Glossary
Notes
Selected Bibliography
Resources
List of Seacrhable Terms
Acknowledgements
About the Authors
Also by Amy Ellis Nutt
About the Publisher
Illustrations
FIG. 1 The Basics of Brain Structure (Brain images courtesy of and with permission from John Detre, MD, and Paul Yushkevich, PhD, University of Pennsylvania). FIG. 2 The “Homunculus” (Artwork by Mary A. Leonard, Biomedical Art and Design, University of Pennsylvania. Brain image courtesy of and with permission from John Detre, MD, and Paul Yushkevich, PhD, University of Pennsylvania). FIG. 3 The Lobes of the Brain (Created by the author, artwork adapted by Mary A. Leonard, Biomedical Art and Design, University of Pennsylvania. Brain image courtesy of and with permission from John Detre, MD, and Paul Yushkevich, PhD, University of Pennsylvania). FIG. 4 Maturing Brain: The Brain “Connects” from Back to Front (A, C: Reprinted from N. Gogtay et al., “Dynamic Mapping of Human Cortical Development During Childhood Through Early Adulthood,” Proceedings of the National Academy of Sciences 101, no. 21 [May 25, 2004], 8174–79, copyright 2004 National Academy of Sciences, U.S.A. B: Brain image courtesy of and with permission from John Detre, MD, and Paul Yushkevich, PhD, University of Pennsylvania). FIG. 5 Multitasking Is Still Not Perfect in the Teen Brain (With kind permission from Springer Science and Business Media and the author: M. Naveh-Benjamin et al., “Concurrent Task Effects on Memory Encoding and Retrieval: Further Support for an Asymmetry,” Memory & Cognition 34, no. 1 [2006], 96, fig. 3A, © 2006). FIG. 6 Anatomy of Neuron, Axon, Neurotransmitter, Synapse, Dendrite, and Myelin (Created by the author, artwork by Mary A. Leonard, Biomedical Art and Design, University of Pennsylvania). FIG. 7A Inhibitory Cells Can Stop Signaling (Created by the author, artwork by Mary A. Leonard, Biomedical Art and Design, University of Pennsylvania). FIG. 7B Excitatory and Inhibitory Synapses (Created by the author, artwork by Mary A. Leonard, Biomedical Art and Design, University of Pennsylvania). FIG. 8 The Young Brain Has More Excitatory Synapses Than Inhibitory Synapses (Courtesy of and created by the author). FIG. 9 Gender Differences in Rate of Cortical Gray Matter Growth (Reprinted with permission from Macmillan Publishers Ltd./Nature Neuroscience: J. N. Giedd et al., “Brain Development in Children and Adolescents: A Longitudinal Study,” Nature Neuroscience 2, no. 10 [1999], 861–63, © 1999). FIG. 10 Long-Term Potentiation (LTP) Is a Widely Used Model of the “Practice Effect” of Learning and Memory (Created by the author, artwork adapted by Mary A. Leonard, Biomedical Art and Design, University of Pennsylvania. Brain image courtesy of and with permission from John Detre, MD, and Paul Yushkevich, PhD, University of Pennsylvania). FIG. 11 New Receptors Are Added to Synapses During Learning and Memory and Long-Term Potentiation (Created by the author, artwork adapted by Mary A. Leonard, Biomedical Art and Design, University of Pennsylvania). FIG. 12 Gray Matter and White Matter Develop Differently Throughout Life (Courtesy of and with permission from Arthur Toga, Institute of Neuroimaging and Informatics, Keck School of Medicine, University of Southern California). FIG. 13 Adolescents’ Synaptic Plasticity Is “Way Better” Than Adults’ (Reprinted from N. L. Schramm et al., “LTP in the Mouse Nucleus Accumbens Is Developmentally Regulated,” Synapse 45, no. 4 [Sept. 15, 2002], 213–19, copyright © 2002 Wiley-Liss, Inc.). FIG. 14 The Developmental Control of the Circadian System (Reprinted from M. H. Hagenauer and T. M. Lee., “The Neuroendocrine Control of the Circadian System: Adolescent Chronotype,” Frontiers in Neuroendocrinology 33, no. 3 [Aug. 2012], 211–29, © 2012, with permission from Elsevier and the author. Additional artwork by Mary A. Leonard, Biomedical Art and Design, University of Pennsylvania). FIG. 15 Ventral Tegmental Area (VTA) Dopamine Neurons from Young Mice Are Able to Fire More Action Potentials Than Those from Adult Mice When Stimulated (Reprinted from A. N. Placzek et al., “Age Dependent Nicotinic Influences over Dopamine Neuron Synaptic Plasticity,” Biochemical Pharmacology 78, no. 7 [Oct. 1, 2009], 686–92, © 2009, with permission from Elsevier. Additional artwork by Mary A. Leonard, Biomedical Art and Design, University of Pennsylvania). FIG. 16 Rates of Alcohol, Cigarette, and Illicit Drug Use from the National Institutes of Health (Courtesy National Institute of Drug Abuse, a component of the National Institutes of Health, U.S. Department of Health and Human Services. Adapted by Mary A. Leonard, Biomedical Art and Design, University of Pennsylvania. Available at http://www.drugabuse.gov/sites/default/files/nida_mtf2012_infographic_1_1000px_3.jpg). FIG. 17 Shared Synaptic Biology of Learning and Addiction (Artwork by Mary A. Leonard, Biomedical Art and Design, University of Pennsylvania). FIG. 18 The Adolescent Brain Responds to Nicotine More Robustly Than the Adult Brain (Reprinted from T. L. Schochet et al., “Differential Expression of Arc mRNA and Other Plasticity-Related Genes Induced by Nicotine in Adolescent Rat Forebrain,” Neuroscience 135, no. 1 [2005], 285–97, © 2005, with permission from Elsevier. Additional artwork by Mary A. Leonard, Biomedical Art and Design, University of Pennsylvania). FIG. 19 Alcohol Decreases LTP (A: Reprinted from T. A. Zhang et al., “Synergistic Effects of the Peptide Fragment D-NAPVSIPQ on Ethanol Inhibition of Synaptic Plasticity and NMDA Receptors in Rat Hippocampus,” Neuroscience 134, no. 2 [2005], 583–93, © 2005, with permission from Elsevier. B: Created by the author, artwork by Mary A. Leonard, Biomedical Art and Design, University of Pennsylvania). FIG. 20 Alcohol Affects LTP in Adolescents More Than in Adults (Reprinted from G. K. Pyapali et al., “Age and Dose-Dependent Effects of Ethanol on the Induction of Hippocampal Long-Term Potentiation,” Alcohol 19, no. 2 [Oct. 1999], 107–11, © 1999, with permission from Elsevier. Additional artwork by Mary A. Leonard, Biomedical Art and Design, University of Pennsylvania). FIG. 21 Increases in Marijuana and Substance Abuse in Teens in the Last Decade (A: Adapted from National Monitoring the Future Study 1997–2011 by Mary A. Leonard, Biomedical Art and Design, University of Pennsylvania. Available at http://files.eric.ed.gov/fulltext/ED529133.pdf. B: Courtesy of Substance Abuse and Mental Health Services Administration, Department of Health and Human Services, National Treatment Episode Data Set 2007, adapted by Mary A. Leonard, Biomedical Art and Design, University of Pennsylvania. Available at http://www.samhsa.gov/data/DASIS/TEDS2k7AWeb/TEDS2k7AWeb.pdf). FIG. 22 Effects of Cannabinoids on Learning. (A: Reprinted by permission from Macmillan Publishers Ltd.: N. Stella et al., “A Second Endogenous Cannabinoid That Modulates Long-Term Potentiation,” Nature 388 [Aug. 21, 1997], 773–78, © 1997. B: Artwork by Mary A. Leonard, Biomedical Art and Design, University of Pennsylvania). FIG. 23 Long-Term Effects of Marijuana on Gray and White Matter in the Teen Brain. (A: Reprinted with permission from M. H. Meier et al., “Persistent Cannabis Users Show Neuropsychological Decline from Childhood to Midlife,” Proceedings of the National Academy of Sciences 109, no. 40 [Oct. 2, 2012], E2657–64, copyright 2012 National Academy of Sciences, U.S.A. B: Reprinted with permission from D. Arnone et al., “Corpus Callosum Damage in Heavy Marijuana Use: Preliminary Evidence from Diffusion Tensor Tractography and Tract-Based Spatial Statistics,” NeuroImage 41, no. 3 [July 1, 2008], 1067–74, © 2008, with permission from Elsevier). FIG. 24 Enhanced Effects of Cocaine on the Behavior of Adolescent Rats. (Reprinted from A.L. Wheeler et al., “Adolescent Cocaine Exposure Causes Enduring Macroscale Changes in Mouse Brain Structure,” Journal of Neuroscience 33, no. 5 [Jan. 30, 2013], 1797–1803a, with permission from Society for Neuroscience. Additional artwork by Mary A. Leonard, Biomedical Art and Design, University of Pennsylvania). FIG. 25 Stress Can Decrease Learning and LTP. (A, C: Artwork by Mary A. Leonard, Biomedical Art and Design, University of Pennsylvania. B: Created by the author, artwork by Mary A. Leonard, Biomedical Art and Design, University of Pennsylvania. D: Reprinted with permission from M. R. Foy et al., “Behavioral Stress Impairs Long-Term Potentiation in Rodent Hippocampus,” Behavioral and Neural Biology 48, no. 1 [July 1987], 138–49, © 1987, with permission from Elsevier. Additional artwork by Mary A. Leonard, Biomedical Art and Design, University of Pennsylvania). FIG. 26 Enhanced Response to Fearful Stimuli in Adolescents Compared with Children and Adults. (Reprinted from B. J. Casey et al., “Transitional and Translational Studies of Risk for Anxiety,” Depression and Anxiety 28, no. 1 [Jan. 2011], 18–28, © 2011 Wiley-Liss Inc.). FIG. 27 Prior Juvenile Diagnoses in Adults with Mental Disorders. (Reprinted from J. Kim-Cohen et al., “Prior Juvenile Diagnoses in Adults with Mental Disorder: Developmental Follow-Back of a Prospective-Longitudinal Cohort,” Archives of General Psychiatry 60, no. 7 [July 2003], 709–17, copyright © 2003 American Medical Association. All rights reserved). FIG. 28 Gender Differences in Brain Connections (Reprinted with permission from M. Ingalhalikar et al., “Sex Differences in the Structural Connectome of the Human Brain,” Proceedings of the National Academy of Sciences 111, no. 2 [Jan. 14, 2014], 823–28, copyright 2014 National Academy of Sciences, U.S.A.). FIG. 29 How Accountable Should Society Hold Adolescents? (Justice John Paul Stevens, United States Supreme Court; Steve Drizin, Northwestern University in Chicago; www.fairsentencingofyouth.org). FIG. 30 A Recap of Brain Development and the Critical Stage of Adolescence (Courtesy of the author).Introduction Being Teen
What was he thinking?
My beautiful, auburn-haired son had just returned home from a friend’s house with his hair dyed jet-black. Despite my inward panic, I said nothing.
“I want to get red streaks in it,” he told me nonchalantly.
I was gob-smacked. Is this really my child!? I’d begun to ask the question often during my fifteen-year-old son Andrew’s sophomore year at a private high school in Massachusetts, all the while trying to be empathetic. I was a divorced working mother of two teenage sons, putting in long hours as a clinician and professor at Boston Children’s Hospital and Harvard Medical School. So if I sometimes felt guilty about the time I spent away from my boys, I also was determined to be the best mother I could be. After all, I was a faculty member in a pediatric neurology department and actively researching brain development. Kids’ brains were my business.
But my sweet-natured firstborn son had suddenly become unfamiliar, unpredictable, and bent on being different. He had just transferred from a very conventional middle school that went through ninth grade, where jackets and ties were the norm, to a very progressive high school. Upon arriving, he took full advantage of the new environment, and part of that was to dress in what you might describe as an “alternative” style. Let’s face it, his best friend had spiky blue hair. Need I say more?
I took a deep breath and tried to calm myself. Getting mad at him, I knew, wouldn’t do either of us any good and probably would only alienate him further. At least he felt comfortable enough to tell me about something he wanted to do before he actually did it. This was an opportunity, I realized, and I quickly seized it.
Instead of damaging your hair with some cheap, over-the-counter dye, what if I take you to my hair guy for the red streaks? I asked him. Since I also was going to pay for it, Andrew happily agreed. My hair stylist, who was a sort of punk rocker himself, got totally into the task. He did a great job, actually—so good that Andrew’s girlfriend at the time was inspired to color her hair in exactly the same black-and-red motif. She attempted this herself, and needless to say had different results.
Thinking back to those days, I realize so much of what I thought I knew about my son during this turbulent time of his life seemed turned on its head. (Was that a compost pile in the middle of his bedroom, or laundry?) Andrew seemed trapped somewhere between childhood and adulthood, still in the grip of confusing emotions and impulsive behavior, but physically and intellectually more man than boy. He was experimenting with his identity, and the most basic element of his identity was his appearance. As his mother and a neurologist, I thought I knew everything there was to know about what was going on inside my teenager’s head. Clearly I did not. I certainly didn’t know what was going on outside his head either! So as a mother and a scientist, I decided I needed to—I had to—find out.
Professionally, I was primarily studying the brains of babies at that time and running a research lab largely devoted to epilepsy and brain development. I was also doing translational neuroscience, which means, simply, trying to create new treatments for brain disorders. Suddenly, however, I had a new scientific experiment and project: my sons. My younger son, Will, was just two years Andrew’s junior. What would I be in for when Will reached the same age as his older brother? There was so much I didn’t get. I had watched Andrew, almost overnight, morph into a different being, yet I knew, deep inside, he was still the same wonderful, kind, bright kid he’d always been. So what happened? To figure it out I decided to delve into the world of research on this somewhat foreign species in my household called the teenager, and use that knowledge to help me and my sons navigate their way more smoothly into adulthood.
The teen brain has been a relatively neglected area of study until only the past decade. Most research dollars in neurology and neuropsychology are spent on infant and child development—from learning disabilities to early enrichment therapy—or, at the other end of the spectrum, on diseases of the elderly brain, especially Alzheimer’s. Up until just a few years ago, the neuroscience of the adolescent brain was underfunded, underresearched, and obviously not well understood. Scientists believed—incorrectly, as it turned out—that brain growth was pretty much complete by the time a child started kindergarten; this is why for the past two decades parents of infants and toddlers, trying to get a jump on their children’s education, have inundated their kids with learning tools and accessories like Baby Einstein DVDs and Baby Mozart Discovery Kits. But the adolescent brain? Most people thought it was pretty much like an adult’s, only with fewer miles on it.
The problem with this assumption is that it was wrong. Very wrong. There are other misconceptions and myths about the teenage brain and teenage behavior that are now so ingrained they are accepted societal beliefs: teens are impulsive and emotional because of surging hormones; teens are rebellious and oppositional because they want to be difficult and different; and if teenagers occasionally drink too much alcohol without their parents’ consent, well, their brains are resilient, so they’ll certainly rebound without suffering any permanent effects. Another assumption is that the die is cast at puberty: whatever your IQ or apparent talents may be (a math or science type versus a language arts type), you stay that way for the rest of your life.
Again, all wrong. The teen brain is at a very special point in development. As this book will reveal, I learned that there are unique vulnerabilities of this age window, but there is also the ability to harness exceptional strengths that fade as we enter into adulthood.
The more I studied the emerging scientific literature on adolescents, the more I understood how mistaken it was to look at the teenage brain through the prism of adult neurobiology. Functioning, wiring, capacity—all are different in adolescents, I learned. I was also aware that this new science of the teenage brain wasn’t reaching most parents, or at least wasn’t reaching parents who don’t have a background in neuroscience as I did. And this was just the audience who needed to know about this new science of the adolescent brain: parents and guardians and educators who are just as perplexed, frustrated, and maddened by the teenagers in their care as I was.
When my younger son, Will, was sixteen, he passed his driver’s test. He’d rarely given me cause to worry, but that changed early one morning. A few weeks after getting his license, he had started to drive himself to school in our 1994 Dodge Intrepid—a big, old, safe car. All seemed to go well. As usual, Will left around 7:30, as school started at 7:55. Off he went. Just as I was walking out the door to go to my job, at about 7:45, I got a call from Will: “Mom, I’m okay, but the car is totaled.” Well, first, I was thankful he had the presence of mind to lead off with telling me he was okay, but I had visions of his car wrapped around a tree. I said, “I’m on my way,” and jumped in my car. As I was approaching the school entrance, I saw the flashing lights of the police cars. What had he done? Well, simply put, he had decided that he could squeeze a left turn into the school entrance in the path of rapidly moving traffic going in the opposite direction. This might have worked if there had been another mother like me driving in the opposite direction who would have shaken her head and slammed on her brakes. But in Will’s case that morning, it was a twenty-three-year-old guy, a construction worker in a Ford F-150 on his way to work. He was no more in the mood to give the right-of-way than Will had been to wait to cross the road. So—the accident happened. It was good to know that 1994 airbags still worked in 2006.