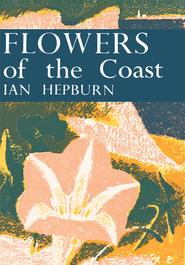
Полная версия:
Flowers of the Coast

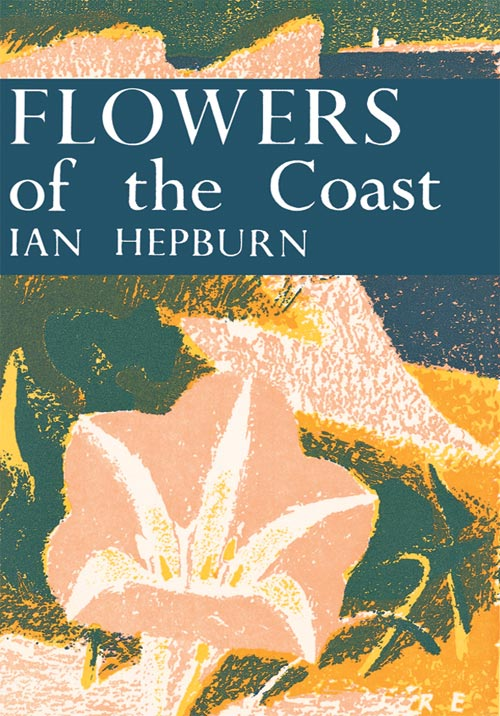
Collins New Naturalist Library
24
Flowers of The Coast
Ian Hepburn
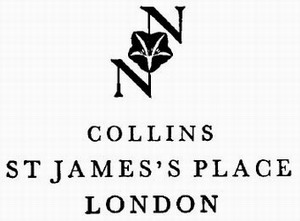
Editors:
JOHN GILMOUR, M.A., V.M.H.
SIR JULIAN HUXLEY, M.A., D.Sc., F.R.S.
MARGARET DAVIES, M.A., Ph.D.
KENNETH MELLANBY, C.B.E., Sc.D.
PHOTOGRAPHIC EDITOR:
ERIC HOSKING, F.R.P.S.
The aim of this series is to interest the general reader in the wild life of Britain by recapturing the inquiring spirit of the old naturalists. The Editors believe that the natural pride of the British public in the native fauna and flora, to which must be added concern for their conservation, is best fostered by maintaining a high standard of accuracy combined with clarity of exposition in presenting the results of modern scientific research. The plants and animals are described in relation to their homes and habitats and are portrayed in the full beauty of their natural colours, by the latest methods of colour photography and reproduction.
toPHYLLISwho loves the sea but is sometimes uncertain of her botany
Table of Contents
Cover Page
Title Page
Editors
Editors’ Preface
Author’s Preface
CHAPTER 1 INTRODUCTORY
CHAPTER 2 THE PHYSIOGRAPHICAL BACKGROUND
CHAPTER 3 SOME ECOLOGICAL CONSIDERATIONS
CHAPTER 4 FORM AND HABIT OF COASTAL PLANTS
CHAPTER 5 SALT-MARSH VEGETATION
CHAPTER 6 STRAND AND FORESHORE VEGETATION
CHAPTER 7 SAND-DUNE VEGETATION
CHAPTER 8 SHINGLE BEACH VEGETATION
CHAPTER 9 VEGETATION OF SPRAY-WASHED ROCKS AND CLIFFS
CHAPTER 10 VEGETATION OF CLIFF-TOPS
CHAPTER 11 VEGETATION OF BRACKISH WATER
CHAPTER 12 THE FLOWERS OF THE COAST
Glossary
Bibliography
Index
The New Naturalist
Plates
Copyright
About the Publisher
EDITORS’ PREFACE

IN ANY civilised country the two types of habitat least altered by man or his domesticated animals are the mountains and the coast. Mountains and Moorlands have already been dealt with in this series by Professor W. H. Pearsall, and, in the present volume, Mr. Ian Hepburn tackles the wild flowers of our coast. Despite the modest disclaimer in his Preface, Mr. Hepburn is particularly well qualified for the task. He is Second Master at Oundle School and one of the leading amateur plant ecologists in the country. He has served on the Council of the British Ecological Society for many years and has published the results of a number of his ecological investigations, including work on the plants of the Northamptonshire limestone and of sea-cliffs. As one would expect, his approach to coastal vegetation is primarily an ecological one, but he has by no means neglected the beauty or botanical interest of the plants themselves, and we feel that he gives in his book a balanced and vivid account of a fascinating subject.
Coastal vegetation has always had a particular attraction for field botanists, and the reasons are not difficult to understand. First, in no other habitat does the flora change so completely in the course of a short walk; one minute there is sand filling our shoes, and marram-grass pricking our legs, and the next a level carpet of sea-lavender lies around us, the dunes are left behind and a grey cliff, with samphire and tree-mallow, lures us farther on. In Britain, probably more than any other country, is this ever-varying quality of the coastline manifest; and the maritime vegetation shows a corresponding richness. Secondly, many of the plants themselves are of great beauty and, in some cases, rarity. The lovely glaucous leaves of the oyster-plant setting off its blue and pink flowers against a Scottish shingle bank, or the autumn squill sprinkling a dry September cliff-turf in Cornwall, spring to the mind—and there are many others. Thirdly, for an ecologist, the coast has a special interest, as nowhere are the succession of vegetation and its dependence on a varying environment so evident, and nowhere have they been more thoroughly investigated. As Mr. Hepburn shows, in an acre or two of salt-marsh are displayed, for those who have eyes to see, most of the more important principles of stable vegetational succession, while on a newly fallen section of cliff, the gradual colonisation of virgin ground can be studied year after year. Lastly, if the environment for the plants is unrivalled in its variety, it is no less so for the humans who study them; a botanist who chooses the British coasts as his hunting ground can enjoy, with a clear conscience, a succession of seaside holidays on what is perhaps the finest coastline in the world.
THE EDITORS
AUTHOR’S PREFACE

IT WAS with considerable diffidence that I, a mere amateur, accepted the invitation of the Editors to write this book. Nor has the subsequent appearance of other books in this series, all written by experts, done anything to dispel this feeling. Nevertheless, I am grateful to them for being so insistent that I should try my hand, for I have greatly enjoyed doing it, and certainly know rather more about the subject than I did when I started!
Ever since I began to take an interest in wild flowers I have always found maritime plants especially attractive. I was lucky enough to go to school within five miles of Blakeney, and first learnt the common seaside plants on the Cley marshes, the Weybourne shingle bank, and sometimes on the historic Blakeney Point itself. Later, I had the good fortune to live for a number of years in north Cornwall, where, in addition to fine stretches of cliffs, excellent sand-dunes and small areas of salt-marsh were within easy reach. Coastal vegetation, therefore, is very much in my blood, and if I have been able, in this book, to convey something of the pleasure I have had in botanising along the coast, I shall be very well satisfied. Now that it is written, my first wish is to get out to the coast once more to start some field-work. Perhaps I may express the hope that others may be similarly stimulated.
I am deeply indebted to many authors, whose books and original papers I have freely consulted. A full list of these, with acknowledgments, appears in the Bibliography. I count myself very fortunate in having persuaded Professor Steers to write the chapter on the physiography of the coast. No one else possesses his detailed knowledge of the whole coastline of Britain, and he should really have written the whole book himself, for he is no mean botanist. I am specially grateful to John Markham for taking so much trouble in obtaining many of the photographs I required—whatever may be said about the text, it will be generally admitted that his pictures are first-class. Finally my grateful thanks are due to John Gilmour for his continual encouragement and advice at all stages.
For permission to reproduce figures appearing in the text acknowledgment is made to the following sources:
Cambridge University Press: Fig. 6, Fig. 9c, and Fig. 11, from The British Islands and their Vegetation (1939) by A. G. Tansley.
G. Bell and Sons, Limited: Fig. 4, Fig. 10, and Fig. 12 from Plant Form and Function (1946) by F. E. Fritsch and E. J. Salisbury.
Royal Geographical Society: Fig. 1 from The Geographical Journal (1937).
CHAPTER 1 INTRODUCTORY

THERE IS NO NEED to possess any great knowledge of botany to see at once that a number of plants grow round our coasts which are never seen inland. It matters little whether you explore a salt-marsh, a sand-dune area, a shingly beach, or some rocky cliffs; in any of these places you can count on coming across some, at any rate, of these purely coastal plants. Moreover, where the conditions are suitable, they often occur in large numbers and are chiefly responsible for the great difference in appearance between seaside and inland vegetation as a whole. In point of fact the characteristic look of the vegetation is often due to the presence of comparatively few coastal species, for along most of the coastline many familiar inland plants can be seen as well. Indeed it is only in salt-marshes, where the whole area is regularly washed by the tides, that practically the whole plant population is composed of maritime species. Most of those growing on sand-dunes, for instance, are also found inland but, since the highly distinctive marram-grass is the dominant species in nearly all areas of blown sand, the vegetation of the whole area acquires its characteristic “coastal” appearance.
It is no good pretending that all these seaside plants are things of beauty. Many of them possess thick fleshy leaves, which do not give them a graceful form, and they often have untidy woody stems. They are apt to have a rather weather-beaten appearance, which is hardly surprising when one remembers the conditions to which they are habitually exposed. No one could suggest that the prickly saltwort (Salsola kali) (Pl. I), the sea-beet (Beta maritima) (Pl. XXXVIII) or the annual glasswort (Salicornia stricta) (Pl. XIII) are attractive to the eye, though like most coastal plants they look interesting because they are unfamiliar. Such charm as they possess is largely associated with the exciting sort of places where they can be found. Yet there are some maritime plants which are as beautiful as any in the land, as the colour illustrations in this book show, and when seen together in a mass produce some glorious colour effects. There are few sights to compare with a West Country cliff in May, when thousands of sea-pinks (Armeria maritima) and sea-campions (Silene maritima) are in full bloom together in their natural rock garden against a background of deep blue sea (Pl. II), or with the startling brilliance of a large sheet of sea-lavender (Limonium vulgare) on some East Anglian salt-marsh in late summer (Pl. 5). In a quieter way, too, the long waving leaves of marram-grass (Ammophila arenaria) on the crests of shifting sand-dunes have an irresistible charm of their own at all times of the year (Pl. III).
Coastal vegetation exhibits many features of particular interest to botanists. Perhaps its greatest attraction lies in the fact that it has, as a whole, been comparatively little altered by man. In a small area like the British Isles there are few places where the clearing of natural forests, the ploughing up of the land, or heavy grazing by domestic animals has not modified or completely transformed the natural vegetation. The thin strip of land bordering the coastline has, however, largely escaped these attentions, since for a number of reasons it does not lend itself easily to agricultural development. Even so, arable farming is frequently carried on quite close to the edges of cliffs, and the higher levels of most salt-marshes are grazed by cattle and sheep. Yet it is not too much to say that practically anywhere along the coast, except in the vicinity of a seaside resort or a port, one can count on finding some patches of maritime vegetation approximately in its natural state. It may be a belt of sand-dunes at the top of a small beach, a little area of salt-marsh around the mouth of a river, or just a collection of foreshore plants on the sand or shingle of the shore, yet they reproduce on a small scale many of the characteristic features of more extensive areas of the same kind of vegetation elsewhere.
Although the coastline has suffered relatively little at the hands of farmers, it is always open to a more serious menace. A justifiable desire of many people to spend their holidays by the sea has led to a great deal of ill-considered building during recent years, in many cases preventing public access to the shore or cliffs. Much irreparable harm has already been done, but the passage of the National Parks Act in 1949 and the setting up of the Nature Conservancy is encouraging evidence that the public has at last woken up to the danger of progressive spoliation of the countryside, and it is to be hoped that a halt may eventually be called to any further defacement. As things are at present, there are fortunately long stretches of unspoilt coast still available for botanical study in many parts of the country, though one could weep over the damage which has been done in the past along some of the more populated parts of the shore.
The coastline provides a number of extremely varied types of habitat in which plants may grow. Salt-marshes, sand-dunes, rocky cliffs and shingle beaches, to mention a few of them, all differ widely from each other in the demands they make upon plants, and each is found to bear a type of vegetation peculiar to its own special conditions. As we shall have occasion to employ the word “habitat” many times in this book it may be as well to make clear at once that it does not merely refer to the place in which a plant is found growing, but includes all those conditions influencing its growth which are connected with the place. In point of fact the main conditions remain remarkably constant in each of the various coastal habitats all over Britain and, as a result, the general type of vegetation found in each of them is strikingly similar wherever it occurs, although there are naturally many local differences of detail.
This similarity can best be appreciated if the botanist, when observing coastal plants, pays special attention to those growing together most frequently in a particular habitat, instead of merely searching for individual (and perhaps rare) species. If he does this, it will soon become apparent to him that many of them habitually occur in well-marked and easily recognised communities. Incidentally, the plant communities found along the coast are, for the most part, simpler in composition than those found inland. This makes them ideal material for study by anyone who is getting beyond the stage of just collecting and identifying plants (a very necessary stage for all field-botanists), and is beginning to take an interest in the vegetation as a whole.
Let it, however, be said at once, for the benefit of the pure plant-hunter, that the coastal regions do in fact provide happy hunting grounds for many relatively rare plants, largely because they have been so little disturbed. This is particularly true of sand-dune areas, which often carry a rich flora, although the rarities found there are usually not confined to the coastal belt. At the same time, I feel strongly that many naturalists would enjoy their botanising even more if they cultivated the habit of looking at the whole vegetation as a unit, noticing how the individual species of which it is composed are grouped, and trying to discover why particular plant communities grow where they do. After all, by doing this, one is not denied the excitement of coming across an unusual plant, and it has the great advantage that the common plants become once more interesting, since their relative frequency is of fundamental importance in determining the nature of the general vegetation. The relation of vegetation to its surroundings is known as plant ecology, and nowhere can a start at this way of botanising be better made than along the coast. In the hope that some of my readers may be stimulated to look at seaside plants in this broader way, the characteristic vegetation of the main coastal habitats is discussed from a general ecological standpoint in the later chapters of this book. With this end in view, I have devoted Chapter 3 to a brief explanation of some of the main principles of plant ecology.
It is important to remember that the coastline never remains completely static, but is continually in process of being either eroded or built up. The ways in which this can take place are discussed in some detail in the next chapter, but it is clear that both erosion and deposition can provide virgin ground, suitable for colonisation by plants. One of the most interesting features of coastal vegetation is that it is often possible to observe a whole series of successive stages whereby these bare habitats acquire a comparatively stable plant-cover, from the first tentative seedlings to such complex communities as are typical of heathland or scrub. This fascinating process in which one community is replaced by another, and this in turn by others, can be witnessed and understood far better along the coast than anywhere else. Unfortunately nowhere is it possible to observe the production under natural conditions of the final or “climax” stage (see page 29) in the development of the vegetation.
But perhaps the best reason of all for studying the flowers of the coast is that by so-doing one visits delightful and exciting places, always within sight and sound of the sea. For my own part, I frankly admit that this was why I became specially interested in seaside plants. Although I have botanised in all sorts of country, and in particular greatly enjoy doing so amongst mountains, I have never experienced anywhere the same variety and pleasure as I have had when looking for flowers along the coast.
A complete list of all the plants growing round the coast of the British Isles would be an extremely long one, but the great majority would be species found equally frequently in quite different habitats far away from the sea. In particular, sand-dunes, cliff-tops, and shingle that is no longer exposed to wave-action, can all support a wide variety of inland plants. It is, however, with those plants which are confined to the coastal belt that we are chiefly concerned in this book, and these fall into two main groups, only the first of which consists of genuine maritime species. This includes practically all the plants found in regularly submerged salt-marshes, and also a number of others occurring in habitats which are commonly exposed to a certain amount of sea-spray, such as beaches and cliffs. The plants belonging to this group are called halophytes (Greek halos=sea salt), and may be roughly described as plants which can live in soils where the water is salt. The main difference between these and normal plants is that their protoplasm (living material) is not destroyed by exposure, either externally or internally, to salt solutions.
One is tempted to divide this group further into “true halophytes,” chiefly confined to salt-marshes and thriving under conditions where the actual water-table remains permanently saline, and what may be called “spray halophytes,” characteristic of habitats exposed to salt spray, but not normally submerged by sea water. Annual seablite (Suaeda maritima) (Pl. 3) and common sea-lavender (Limonium vulgare) (Pl. 5) are examples of true halophytes, while spray halophytes are exemplified by samphire (Crithmum maritimum) (Pl. 10) or sea-rocket (Cakile maritima) (Pl. XVI). We hardly know enough yet about the requirements of many of these plants, however, to make such a distinction and it is therefore inadvisable to carry it far. Some true halophytes, for instance, appear actually to require the presence of salt in their root-water for their full development, but probably all spray halophytes and certainly some “true” ones (e.g. thrift) can grow equally well in ordinary garden soil, provided that competition from taller and faster-growing plants is excluded. Indeed the absence of competition from other plants, which are unable to endure the conditions, is the chief reason for the abundance of spray halophytes on exposed cliffs and similar habitats. Since the term “halophyte” is used to describe plants of such differing requirements, it is probably best to consider that the one essential feature they have in common is that they can tolerate a certain amount of salt water, rather than that they actually require its presence. Moreover, it should not be forgotten that sea-water contains small quantities of many other salts besides sodium chloride, and it is quite possible that these may also have important effects on the growth of plants, which we do not at present understand. The majority of halophytes are perennials and often develop extensive woody rootstocks. But their most obvious physical feature is usually their fleshy or succulent leaves, a characteristic which appears to be closely connected with the absorption of salt water, since it is also shown by a number of inland plants when they grow near the sea or inland salt areas.
The second group of plants confined to the coastal belt consists of those found only on sand-dunes. There is, of course, nothing inherently maritime about a sand-dune, but it so happens that in the British Isles the main dune areas are coastal. The largest areas of blown sand in the world are found in desert regions far from the sea, and in North America extensive “coastal” dunes exist round the Great Lakes, where the water is fresh. Even in this country considerable patches of blown sand, carrying some typical dune plants, can be seen in the Breckland. All plants capable of establishing themselves in mobile sand bear a general resemblance to each other wherever they occur, and the majority of them are known as xerophytes (Greek xeros=dry). Most of them possess unusually extensive roots or rhizomes (buried stems), and their leaves are often adapted in some way or other to cut down excessive loss of water from them. Many xerophytes have succulent leaves and stems, similar to those belonging to halophytes. This likeness, is, however, largely accidental and is due in this case to the development of water-holding cells, called collectively “aqueous tissue” (see page 47), for the purpose of storing water. Xerophytes are by no means confined to dune areas in Britain but may also be found in other dry habitats inland. Those confined to dunes, besides possessing an unusual ability to withstand drought, are also able to deal with the problem of occasional burial in loose sand by their exceptional powers of sending up new shoots as soon as they are covered over—marram-grass (Fig. 10) possessing this power to a quite remarkable degree. Other familiar examples of dunc-xerophytes are sea-holly (Eryngium maritimum) (Pl. 1) and sea-sandwort (Honckenya peploides). Shingle beaches and dry cliffs are other coastal habitats where plants of this group commonly occur. The characteristic form and habit adopted by both halophytes and xerophytes is discussed in greater detail in Chapter 4.
The remainder of the plants to be seen in the coastal belt are too numerous and varied for it to be worth while attempting further classification. Practically all types of inland vegetation are represented somewhere along the coast, although woodland is virtually absent from all exposed situations and is confined to the borders of sheltered estuaries or to deep valleys (or coombes) which have been cut through cliffs, where sufficient protection from the wind is provided. Typical marsh plants, for instance, grow in the wet “slacks” sometimes found between ranges of sand-dunes (Pl. XXII), and in north-west Scotland and Ireland, alpine plants, characteristic of the higher mountains in Britain, occur near sea-level on the cliffs and beaches just as they do farther north in sub-arctic regions. Nor is it surprising that a large number of common plants, usually associated with dry waste-places of all kinds, find a home on the older stabilised dunes and shingle beaches, particularly shallow-rooted annuals, which can pass through their life-cycle during the winter and spring, before the advent of the summer droughts.